Why “∆G = ∆H – T∆S” is the most important equation in biochemistry
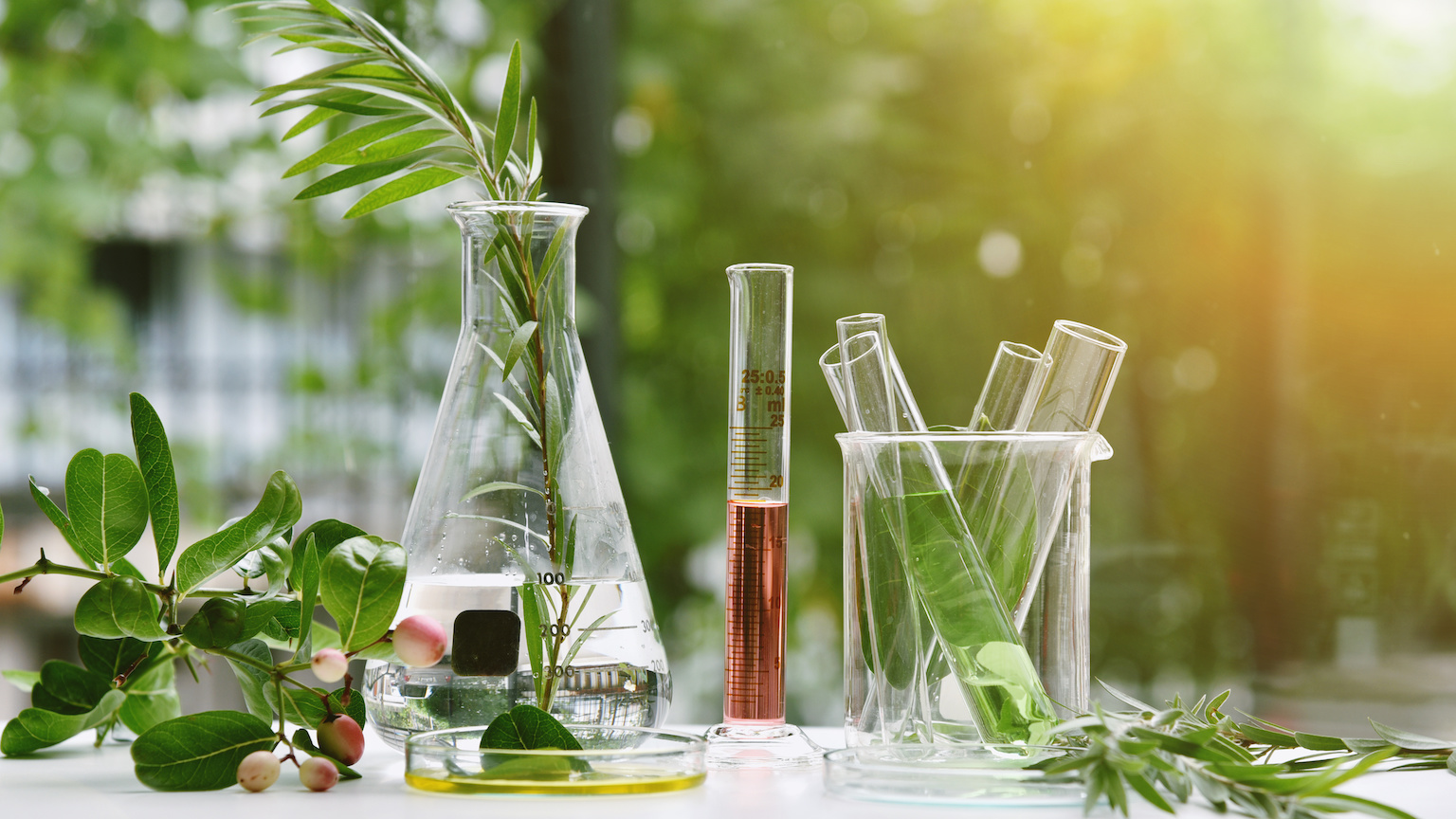
- Biochemistry is the study of biologically relevant chemical reactions, mainly those involving carbon-containing molecules.
- “∆G = ∆H – T∆S” is the most important equation in biochemistry because it reveals that spontaneous chemical reactions — those that occur all by themselves without external intervention — must release free energy (∆G).
- Cells use the free energy released by spontaneous chemical reactions to drive non-spontaneous chemical reactions, which require an input of free energy. If cells could not “couple” reactions in this way, life itself would not be possible.
Biochemistry is the study of biologically relevant chemical reactions — mainly, those involving organic (carbon-containing) compounds. One of the main themes of biochemistry is understanding which chemical reactions occur in living organisms and why. And the major equation that describes this is: ∆G = ∆H – T∆S.
In English, that says “the change in free energy (G) equals the change in enthalpy (H) minus temperature (T) times the change in entropy (S).” Does that clear things up? Probably not.
∆G = ∆H – T∆S
To understand this extremely abstract equation, let’s break it down. Anytime there is a delta (∆), that means “change in.” For instance, if we began with seven cookies and now only have two left, the change in (∆) cookies is -5. The reason we measure change (∆) is because the underlying value might be difficult if not impossible to measure. With the exception of temperature (T), none of the other terms can be measured directly. We can only measure changes.
Free energy (G) refers to energy that is available to do useful work. When you run your laptop or smartphone, the battery is not able to do useful work with all of the stored energy. How do we know? Because those electronic devices get hot. That’s not supposed to happen in an ideal world; it’s wasted energy. But there is nothing we can do about it because we don’t live in an ideal world. (More on that in a moment.) As a result, the change in free energy (∆G) is always less than the total amount of energy that could have been extracted for useful work.
Enthalpy (H) is a slightly odd concept that chemists invented to help them understand energy changes during chemical reactions. The change in enthalpy (∆H) is essentially the change in heat. (It is slightly more complicated than that, as it also includes pressure-volume work. Thankfully, that is often negligible and can be ignored.) Chemical reactions either absorb heat (and become colder to the touch) or release heat (and become warmer to the touch), and the change in enthalpy (∆H) measures this.
T∆S, the final term, represents the product of temperature (T) and the change in entropy (∆S). Remember that ideal world of perfect batteries that we don’t live in? The second law of thermodynamics tells us that entropy (S, which can be thought of as “waste energy” or “disorder” or “chaos”) always increases in the Universe. You can think of entropy as a sort of “tax” that the Universe charges for every energy transfer. T∆S, which represents the quantity of this “tax,” must be subtracted from ∆H.
Let’s try again to restate the equation ∆G = ∆H – T∆S in plain English: The change in the amount of energy available to do useful work (∆G) equals the change in heat (a form of energy transfer) of a chemical reaction (∆H) minus some ridiculous “tax” that the Universe charges (T∆S).
∆G determines whether a chemical reaction can occur
Great. What does that have to do with life? So glad you asked!
Life is complex, and it requires many chemical reactions that do not occur “spontaneously.” A spontaneous reaction occurs with no intervention. If left to its own devices (that is, there is no input of energy), the chemical reaction will occur by itself. Note that this has nothing to do with speed. A spontaneous reaction can be very fast or incredibly slow. Corrosion due to rust formation is spontaneous, but it takes a long time.
For the cells of your body to survive, they need to perform both spontaneous and non-spontaneous reactions. (An example of a non-spontaneous reaction is building a protein.) The way that a cell can perform non-spontaneous reactions is by “coupling” a spontaneous reaction (which is energetically favorable and releases free energy) to a non-spontaneous reaction (which is energetically unfavorable and requires an input of free energy). As long as the overall process results in a net release of free energy (by convention, we consider this a negative ∆G value), the reaction can proceed.
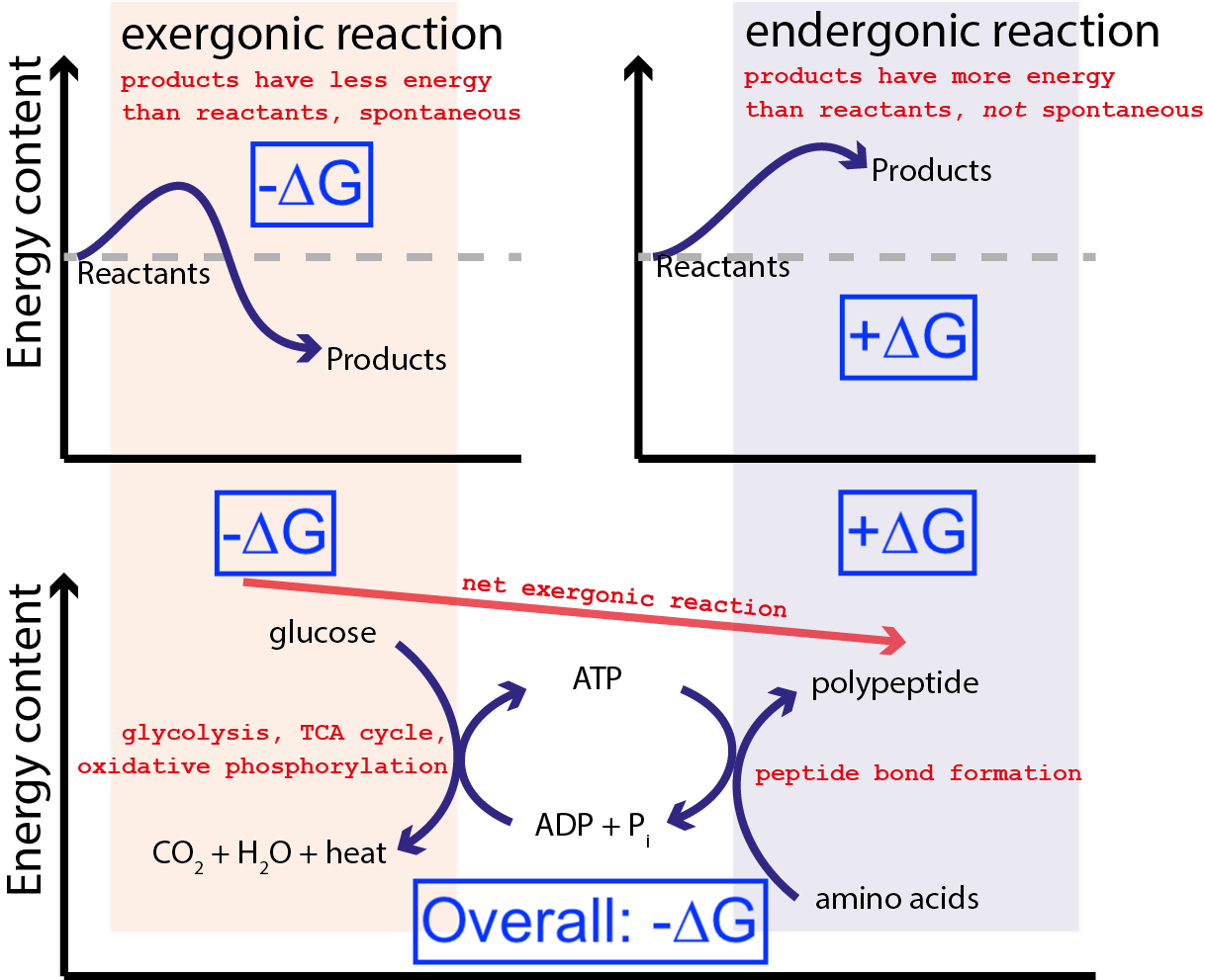
The above figure shows a very typical series of chemical reactions in a cell. A cell can extract free energy from glucose (-∆G) to produce an intermediate and highly energetic molecule (called ATP) that drives protein synthesis, which requires an input of free energy (+∆G). Because the overall process has a net release of free energy (-∆G), the reaction can proceed.
If our cells did not have the ability to drive non-spontaneous, energetically unfavorable chemical reactions (+∆G) using spontaneous, energetically favorable chemical reactions (–∆G), life could not exist. That is why ∆G = ∆H – T∆S is the most important equation in biochemistry.
Note: This is the end of the article. But hardcore biochemistry enthusiasts can feel free to keep reading!
Bonus material:
∆G = ∆G°’ + RT ln( [C][D] / [A][B] )
As is usually the case, the science is actually far more complicated than that. Consider a generic chemical reaction of the form: A + B ⇌ C + D, where A and B are reactants and C and D are products. The actual most important equation in biochemistry is an alternate definition of the change in free energy (∆G):
∆G = ∆G°’ + RT ln( [C][D] / [A][B] )
This equation defies a quick and easy explanation, but the general gist is that the change in free energy (∆G) is dependent on the inherent nature of the chemical reactants and products (∆G°’) plus a constant (R) times temperature (T) times the natural logarithm (ln) of the product of the concentrations of C and D (that is, [C] times [D]) divided by the product of the concentrations of A and B (that is, [A] times [B]).
∆G°’, known as the standard free energy change at pH 7, is a value that is calculated when all of the products (C and D) and reactants (A and B) are present initially at a concentration of 1M (1 mole/liter) at 1 atmosphere of pressure at 25° C. Under these “standard conditions” — artificial conditions only found inside of a test tube in a laboratory — the concentration of the reactants ([A] and [B]) and of the products ([C] and [D]) will always stabilize at very specific values that depend on the nature of the chemical reaction. The value K’eq is a constant that is unique to every chemical reaction, and it is calculated by multiplying the final concentration of the products ([C] times [D]), then dividing by the product of the concentration of the reactants ([A] times [B]).
K’eq = [C][D] / [A][B]
Because K’eq is a constant, R is a constant, and temperature (T) is defined to be 25° C under standard conditions (which means it also is a constant), the value ∆G°’ is itself a constant that is unique to every chemical reaction.
∆G°’ = -RT ln(K’eq)
But it gets even more complicated. Whether a reaction is spontaneous or non-spontaneous depends on ∆G, not ∆G°’. The conditions inside of a cell are not standard conditions. The reactants and products are never at a concentration of 1M (1 mole/liter) each, and the temperature is not 25° C. So, calculating ∆G requires taking into account those considerations, which is how we arrive at the formula shown above:
∆G = ∆G°’ + RT ln( [C][D] / [A][B] )
Let’s try translating this into English: The change in free energy available to do useful work (∆G) equals the change in free energy that is characteristic of the specific reactants and products used under artificial laboratory conditions (∆G°’) plus a constant (R) times the actual temperature (T) times the natural logarithm (ln) of the product of the actual concentrations of C and D found inside of a cell (that is, actual [C] times actual [D]) divided by the product of the actual concentrations of A and B found inside of a cell (that is, actual [A] times actual [B]). If after all that ∆G is negative, the reaction is spontaneous.