Dark matter search: Are scientists looking in the wrong direction?

- Dark matter, believed to account for most of the Universe’s mass, remains undetected despite 50 years of searching.
- The leading candidate, WIMPs, has not been observed in experiments, prompting questions about the direction of dark matter research.
- New measurements suggest scientists may need to explore alternative candidates like axions to solve this cosmic mystery.
Dark matter is thought to be the most prevalent form of matter in the Universe, comprising about five times as much mass as the ordinary matter found in stars and galaxies. There’s only one problem: Despite half a century of effort, scientists have found only indirect evidence of its existence. Now, a measurement from an ultra-precise new facility is hinting that scientists may have been looking in the wrong direction.
Something isn’t right in the Universe. Galaxies move too quickly and spin too rapidly to be explained by the observed matter in the cosmos and the accepted laws of physics. The most popular explanation for these curious observations is that the Universe is permeated by a large amount of unseen — and unseeable — form of matter. This matter, tentatively called dark matter, can interact gravitationally with ordinary forms of matter, but dark matter neither emits nor absorbs light.
While several candidate forms of dark matter have been proposed and rejected, since the late 1990s the scientific community has settled on a favored candidate of dark matter called a WIMP (Weakly Interacting Massive Particle). If WIMPs are real, the consensus is that they are stable subatomic particles, electrically neutral, with a mass in the range of perhaps 100 to 100,000 GeV. (A GeV is an energy unit that is connected to mass through Einstein’s equation E = mc2. For context, a proton has a mass of about 1 GeV.)
According to popular theory, WIMPs cannot be seen, although they do exert gravity. Unlike ordinary matter, which coalesces into stars and planets, WIMP dark matter is organized as vast clouds of “gas” that surround and permeate galaxies, including our own Milky Way. If such clouds exist, a gas of dark matter can be found in the Solar System and forms a “WIMP wind” that is constantly passing through Earth. Depending on the mass of individual WIMP particles, if you hold up your fist, there is perhaps one WIMP particle in that volume.
Scientists have looked for this WIMP wind without success, despite a series of increasingly sensitive detectors. Since the mid-1980s, detectors have increased their sensitivity a million-fold. One of the current, world-class, detectors is called LUX-Zeplin (LZ). It uses 10 tons of liquid xenon, chilled to approximately -150 °F (-100 °C), and is located about 4850 feet underground (1478 meters) at the Sanford Underground Research Facility (SURF) in an abandoned gold mine.
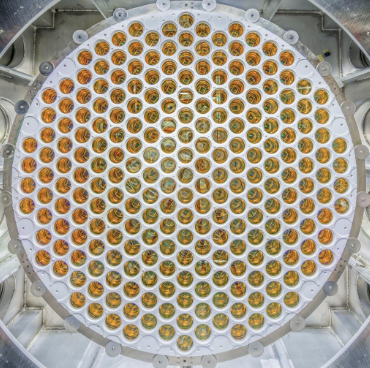
This depth shields the detector from a constant barrage of cosmic rays from space, which can mimic dark matter signals. Only seven of the 10 tons of xenon in the central core of the detector are used in subsequent analyses; events in which signals are seen in the three tons of liquid xenon that form a shell around the inner core are rejected. This ensures that any possible WIMP detections are well-measured.
The LZ experiment waits for a WIMP particle to pass through the detector and hit a xenon atom. The prediction is that debris from that collision will pass through the apparatus and leave a detectable signal. After running for 280 days (out of an expected 1,000), the LZ scientists did not detect the passage of WIMPs through their detector. They recently reported their results at the TeVPA and LIDINE conferences. A scientific paper is under preparation, though some caution is warranted because the announced results have not yet been peer-reviewed. (Early release of measurements is common for large experiments like LZ, and it is very rare that peer review significantly changes the measurement as it is initially reported.)
The measurement announced by LZ reflects a five-fold improvement in the range of WIMP masses and interaction probabilities that are ruled out. While the existence of WIMPs remains a possibility, the current measurement makes their existence less likely. There remains one range of masses that LZ was unable to rule out. The analysis they reported did not consider WIMP masses below 9 GeV.
Given the range of masses favored by most WIMP theories (100 – 100,000 GeV), if it turns out that dark matter is so light, it is possible that dark matter isn’t a WIMP at all. LZ is continuing to analyze its data. The fact that they avoided masses below 9 GeV isn’t because they don’t have data down there; rather, it’s that low mass analyses are very difficult and will require more care before any results are available.
Future, larger, liquid xenon experiments will be able to make even more sensitive measurements, however, there is a cap on how much WIMP experiments can be improved. You see, Earth is constantly being bathed by particles called neutrinos. These neutrinos originate both from nuclear reactions in the sun and from cosmic rays from space. Effectively, Earth exists in a neutrino fog.
Unfortunately, neutrino interactions in WIMP detectors look much like dark matter interactions, so when dark matter experiments are sensitive enough to detect this neutrino fog, it will become impossible to detect dark matter interactions. It will be like trying to hear a whisper at a rock concert.
A detector with sensitivity about 10 times better than LZ would be able to detect the neutrino fog. It will likely take 10 to 15 years to develop detectors with that level of sensitivity. Once achieved, traditional WIMP searches will no longer be possible.
Does this mean we are approaching the end of the road for dark matter searches? Not at all. After all, the unexpected motion of galaxies needs an explanation. It could be that dark matter is some form of matter that isn’t a WIMP. Indeed, some scientists are turning their attention to a much lighter dark matter candidate, called an axion. Axions, like WIMPs, have not been detected, but axion detection requires very different technologies than WIMP particles. The search for dark matter will continue.