3 Big Lessons We Can All Learn On The Hubble Space Telescope’s 30th Anniversary
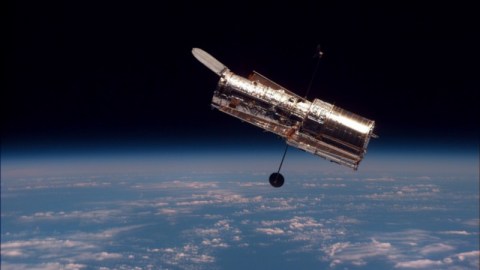
Over the past 30 years, we’ve revolutionized what we know about the Universe. But we couldn’t have done it without these lessons.
On April 24, 1990, the Hubble Space Telescope rocketed into low-Earth orbit, where it’s remained for 30 years. It was serviced four times throughout its lifetime, correcting flaws, repairing and replacing on-board equipment and installing improved instruments. Even today, 30 years after its launch and 11 years after its final servicing, it remains the greatest space-based optical observatory in all of human history.
It’s easy to look back at the myriad of discoveries made with the Hubble Space Telescope and marvel at how they’ve revolutionized our view of the Universe, from the Solar System to the most distant reaches of deep space. But perhaps even more important than any specific discoveries are these three lessons, 30 years on, that illustrate how we’ve used this spectacular piece of equipment to advance our fundamental understanding of the cosmos.
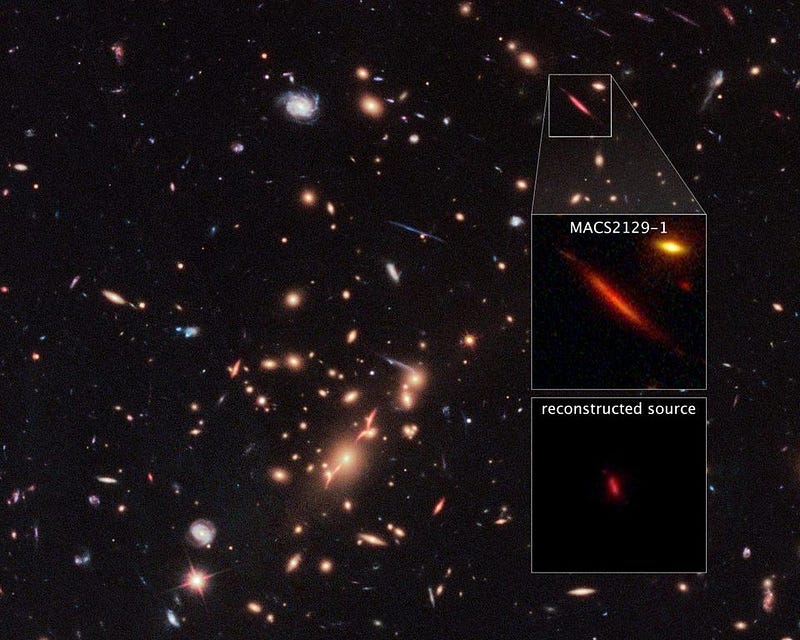
1.) Whenever you can, look as you’ve never looked before. This was the fundamental motivation for even building and flying this observatory. Here on Earth, astronomers have no choice but to contend with our atmosphere. As transparent as it is to visible light, it’s still like viewing the Universe from the bottom of a swimming pool. Clouds, particulates, and even just the distortive effects of turbulent airflow all make it incredibly difficult to determine fine details about the Universe.
Even with the tremendous advances that adaptive optics have made over the past three decades, even with much larger telescopes available on the ground compared to Hubble’s modest 2.4-meter primary mirror, there are still enormous classes of observations that Hubble is uniquely suited for. In other words, it’s capable of viewing the Universe to greater precisions, depths, and in unique wavelength bands compared to any other observatory.
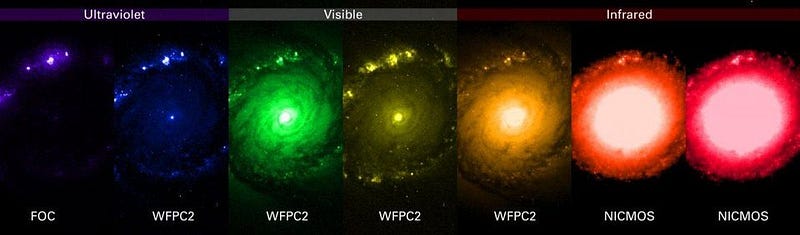
This is, in many ways, the most important reason why Hubble is so valuable. It allows us to look at the Universe in a way that reveals things that no other observatory has ever revealed before. In particular, Hubble’s capabilities enabled:
- the highest-resolution ultraviolet observations ever, including unprecedented ultraviolet spectroscopy (which the atmosphere forbids from the ground),
- the ability to resolve objects as small as 0.05 arc-seconds, or 1/72,000th of a degree, without any adaptive system or software processing,
- infrared wavelengths that go out to almost 2,000 nanometers, or three times the limit of the longest-wavelength light human eyes can see (and that cannot be well-observed from the ground due to atmospheric absorption),
- and the ability to perform long-exposure astronomy due to the low noise levels from space, enabling deep-field views as never before.
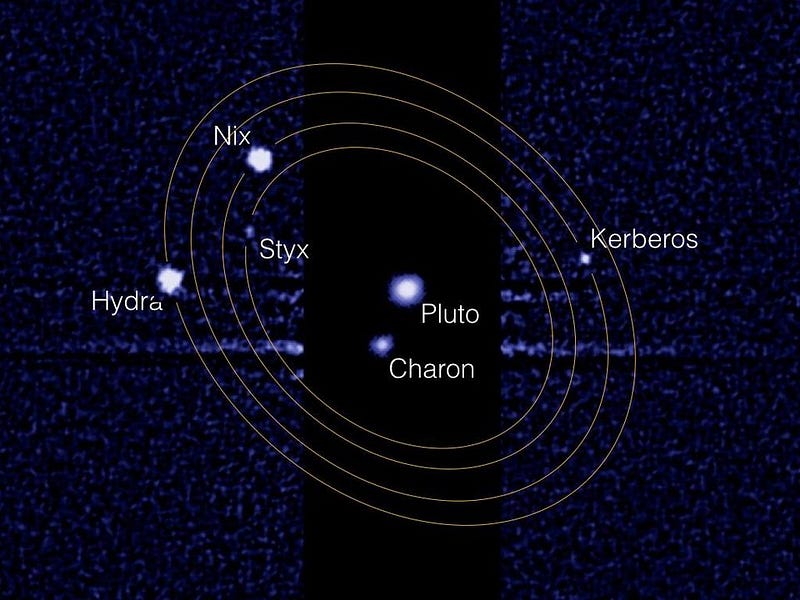
Sure, we’ve reached the limits of what Hubble can do on these frontiers, but these new limits are many times better than the pre-Hubble limits. Any time you push to fainter limits, greater wavelength coverages, wider observational fields-of-view, and higher resolutions, you’re able to see new objects and new details in those objects, eclipsing our previous knowledge sets.
Sometimes, the act of looking alone is enough to reveal new truths about the Universe. Hubble discovered:
- four new Plutonian moons,
- the first directly-imaged exoplanet,
- dozens of protoplanetary disks around newly forming stars,
- hundreds of new supernovae that revealed the expansion history of the Universe,
- and the most distant galaxy ever found,
among many others. Whenever you build a new tool, you unlock the capability of seeing the Universe as never before. With the James Webb Space Telescope, WFIRST, and a slew of new proposals on the horizon, humanity is poised to take the next great leap into the distant Universe.
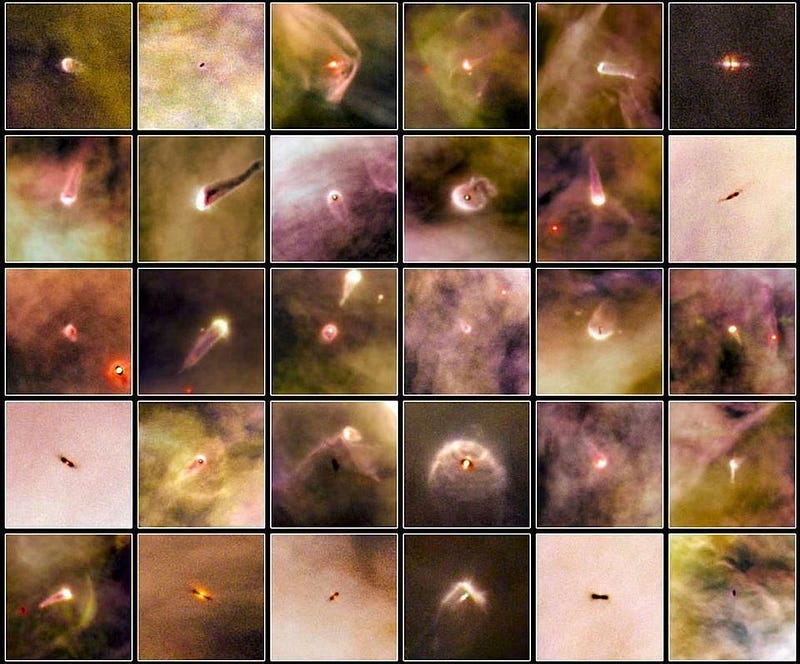
2.) Always follow the evidence, no matter where it leads. This is one of the most underappreciated lessons in all of science, and it particularly applies to the Hubble Space Telescope. We can see this by looking at the scientific motivation for even building and flying this telescope in the first place. It’s literally right there in its name: it’s named the Hubble Space Telescope not because it was to honor Edwin Hubble, but because its main science goal was to measure how quickly the Universe was expanding: to measure the Hubble constant.
The telescope was designed with the capabilities to perform those measurements, observing many different properties of galaxies to simultaneously determine their brightness, size, redshift, and a myriad of other properties. After 10 years of operations, they released the results of the Hubble Space Telescope Key Project, and did it: they successfully determined the rate at which the Universe was expanding.
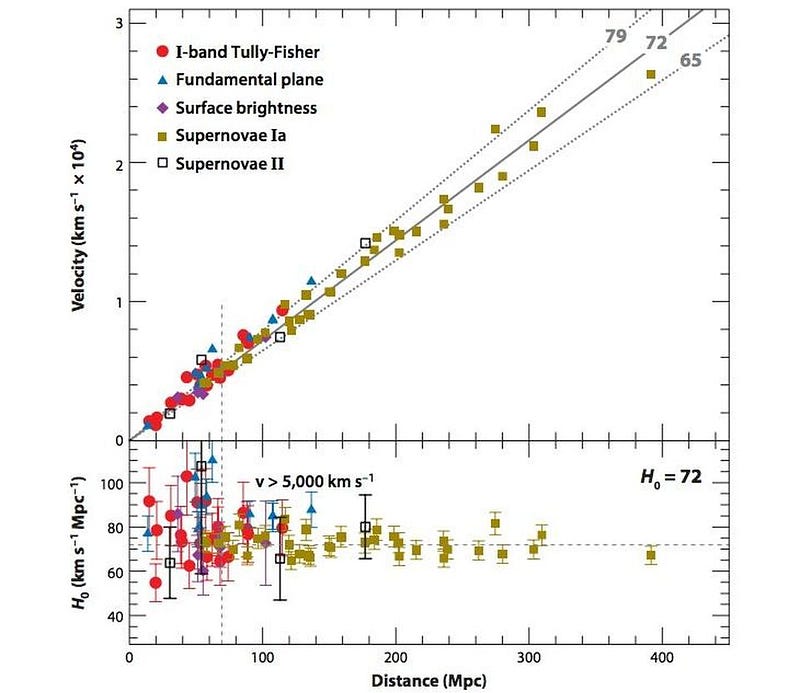
But along the way, Hubble taught us a whole slew of lessons that we weren’t anticipating. It helped determine that the Universe wasn’t just expanding, but that the expansion was accelerating: our Universe was dominated by dark energy. Even today, most of the data informing our best measurements of that accelerated expansion come from the Hubble Space Telescope.
We discovered how galaxies grew and evolved over cosmic time, determined when star formation peaks, measured precisely when in the distant past the Universe became fully ionized, showed us unprecedented details about how stars die, and even helped us learn what the age of the Universe is. It gave us spectacularly large numbers of galaxies that were — just by chance — serendipitously aligned with large intervening masses, producing images of gravitational lenses that are as spectacular as they are scientifically valuable.
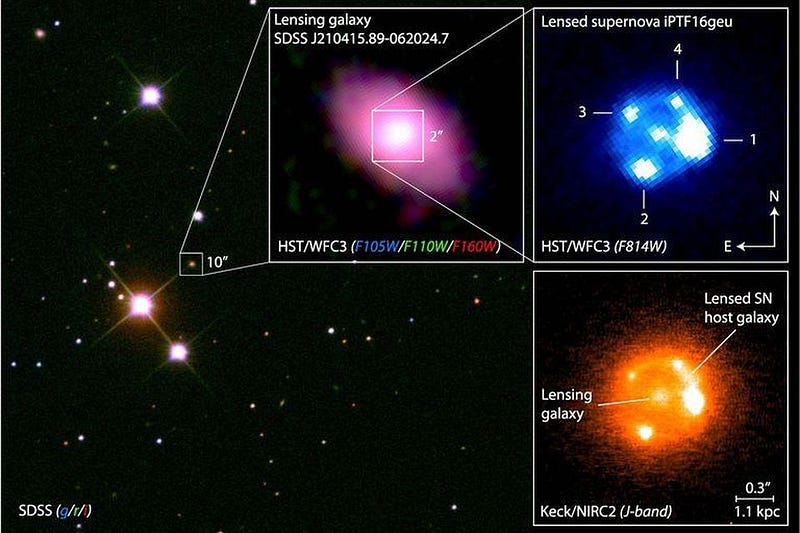
In every single instance, we had theories and models that fit all the evidence we had at the time prior to Hubble concerning each of these scientific issues. After the Hubble data came in, the leading scenario concerning every single one of these phenomena needed to be revised in some way, from small tweaks to complete overhauls.
We were able to push the frontiers in ways that they had never been pushed before, and that led to new observations, new data, new results, and — in many cases — new and surprising conclusions. We built the Hubble Space Telescope with a particular science goal in mind, but its capabilities enabled us to explore corners of the Universe we didn’t even know existed at the time of the telescope’s design. We followed the evidence wherever it led us, and the Universe revealed secrets we didn’t even fathom it could possess.
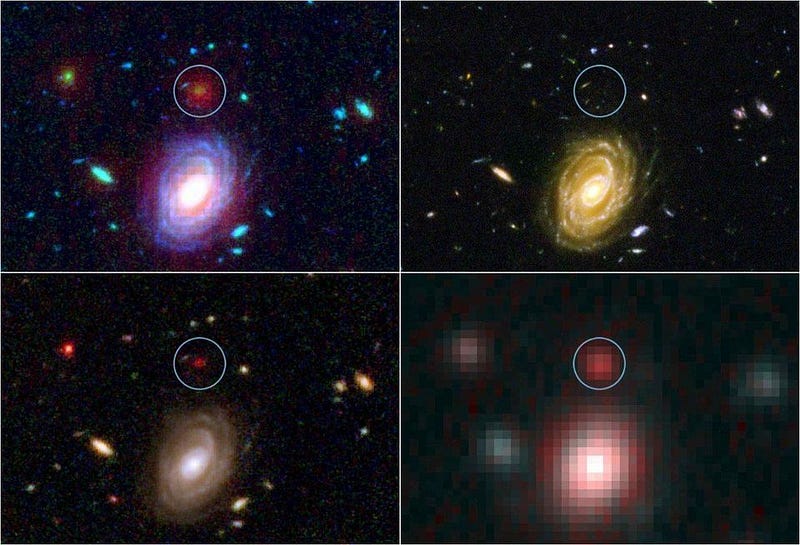
3.) There is a ‘right way’ to be wrong. Being wrong is one of the most important components of any scientific advance. You have a prevailing theory, that theory makes predictions, those predictions translates into observational or experimental tests, and you use the best investigative tools at your disposal to perform those tests. When you get your results, you never know what you’re going to find. Possibilities include:
- they’re consistent with what the leading theory predicted, at least, within the errors,
- they’re inconsistent with the prevailing theory’s predictions to some significant degree,
- they’re consistent with a number of plausible alternatives, while excluding or disfavoring other alternatives,
- or perhaps they point away from the consensus line-of-thought entirely, pointing to the need for a new direction or a new set of considerations.
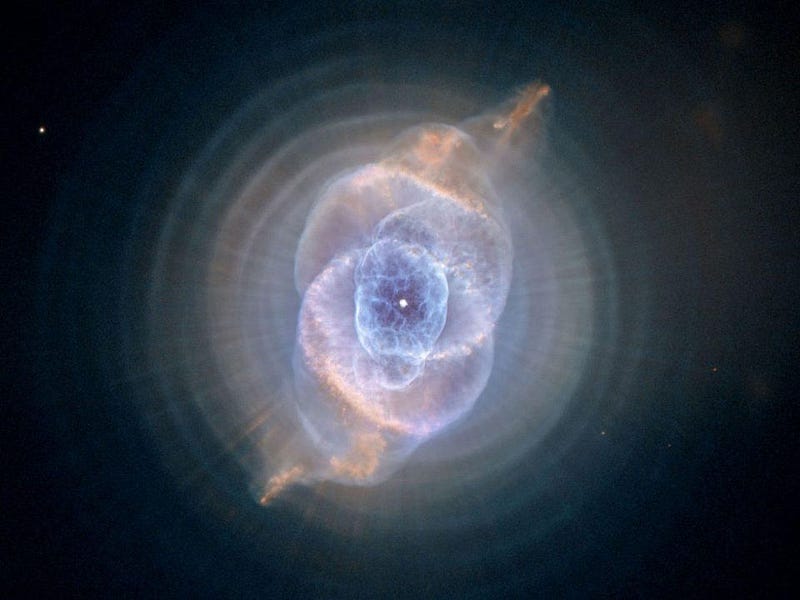
There are two paths to take that are very tempting, but are both scientifically dubious and even dangerous. One is to assume that the leading theory is correct, and to toss away outlier data until your results conform with what you expected. Another is to trust in your data entirely, irrespective of any other concerns, and to draw a speculative, even fantastic conclusion based on the novel results you’ve obtained.
But the responsible course of action is to analyse your new data as responsibly as you can, as though you didn’t know what the outcome implied, and then to draw your conclusions based on the full suite of data available: all of your new data plus all of the other data, including from complementary methods, that other researchers have collected. Only by synthesizing all of the relevant information together can we ever hope to form a fully consistent picture of our physical reality.
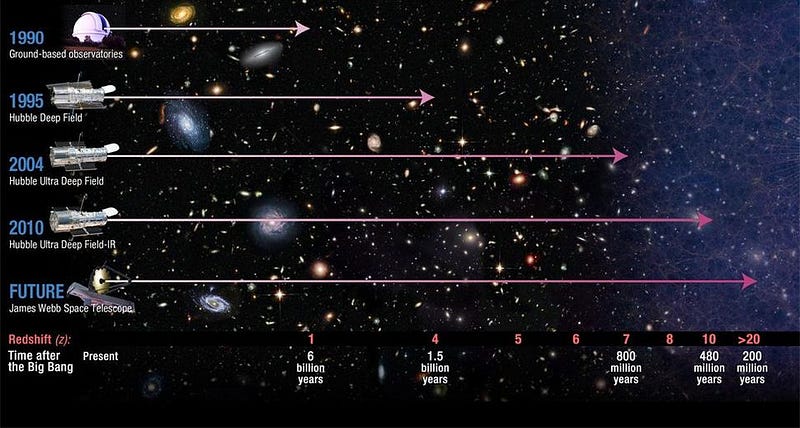
Before Hubble, we did not know how fast the Universe was expanding. We did not know its age; we did not know how much matter was in it; we did not know whether its ultimate fate was to recollapse or expand forever. We did not know when stars and galaxies first formed, what the earliest ones were like, or the details of how stars were born and died. We did not even know whether there were planets in solar systems beyond our own.
30 years later, we have the answers to all of these questions, largely thanks to scientific contributions made using this one astronomical observatory. New questions have arisen in their place, as pushing the cosmic frontier back to new depths always leads to the discovery of novel phenomena that themselves require an explanation. The cosmic frontier is truly endless in this regard. May we always remain curious enough to investigate and solve whatever mysteries the Universe lays before us.
Ethan Siegel is the author of Beyond the Galaxy and Treknology. You can pre-order his third book, currently in development: the Encyclopaedia Cosmologica.