Why the Earth has a liquid core
Under the tremendous pressure and at the incredible temperatures of the Earth’s deep interior, there’s a thick layer of liquid: our outer core. But why is it so?
“If you ever drop your keys into a river of molten lava, let ‘em go, because, man, they’re gone.” –Jack Handey
Take a look at our home planet, Earth, and one of the things you’ll notice is that over 70% of the surface is coated in water.
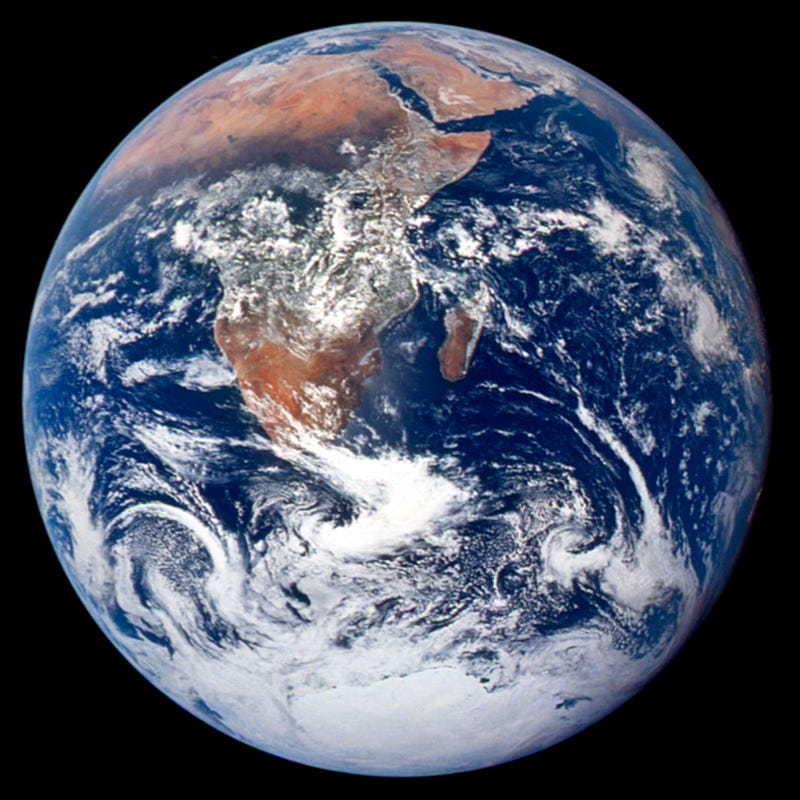
We all know why this is, of course: it’s because the Earth’s oceans float atop the rocks and dirt that make up what we know as land.
This concept of flotation and buoyancy — where the less dense objects rise above the denser ones, which sink to the bottom — does much more than just explain the oceans.
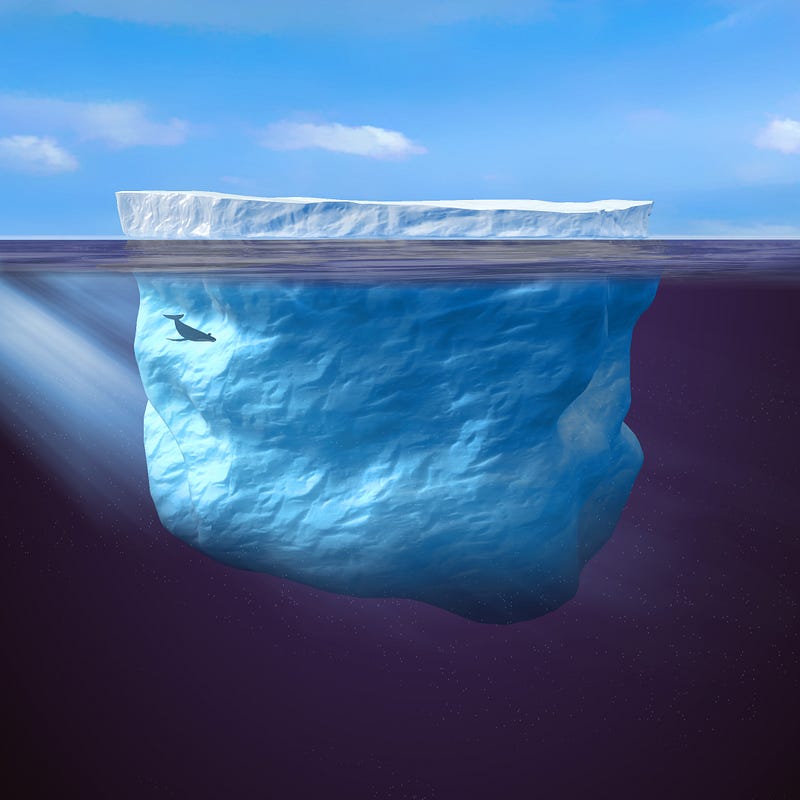
This same principle explains why ice floats on water, why a helium balloon rises through the atmosphere or why stones sink to the bottom of a lake, the last one of which is that the less dense water rises around the stone. This same principle — of buoyancy — also explains why the Earth is layered the way it is.
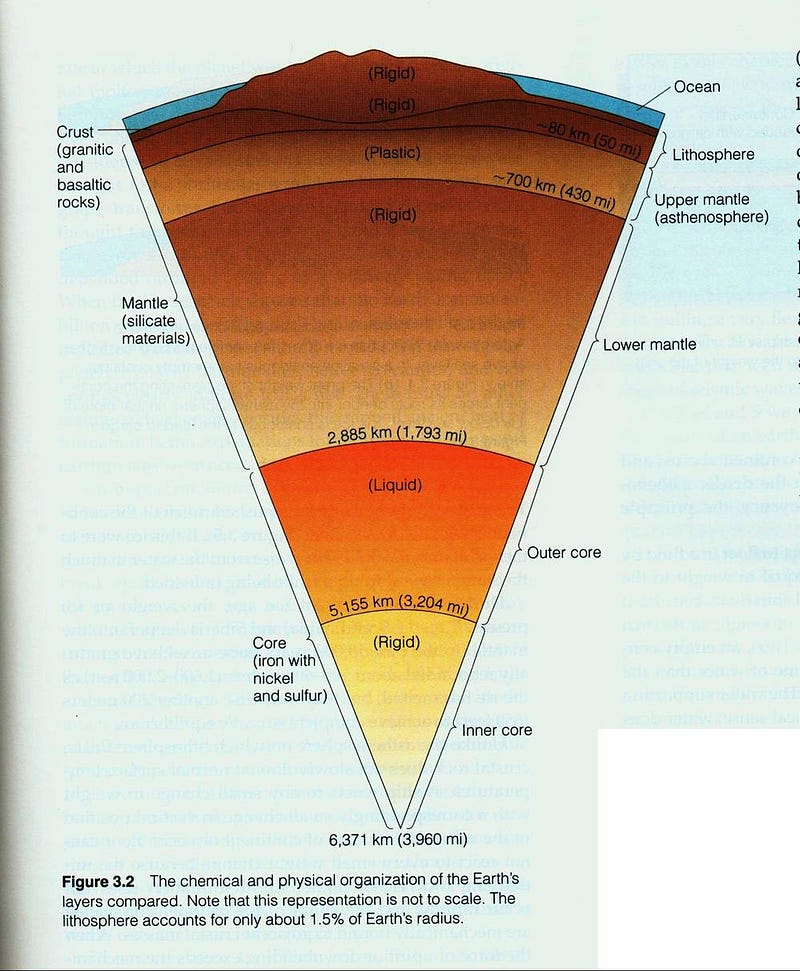
The least dense part of the Earth, the atmosphere, floats atop the watery oceans, which in turn float atop the Earth’s crust, which lies above the more dense mantle, which itself cannot sink down into the densest section of the Earth: the core.
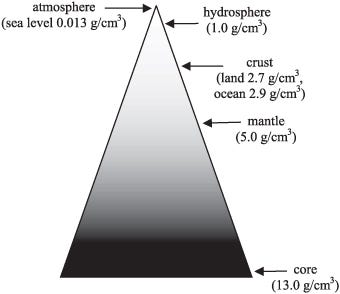
Ideally, the most stable state the Earth could conceivably be in is one that was perfectly layered like an onion, with the densest elements all towards its center, with each outward layer progressively made up of less dense elements. In fact, each earthquake that occurs on Earth is actually the planet moving one very tiny step closer towards that ideal state, as our rate-of-rotation speeds up slightly in the aftermath of each one.
And this picture of our world, layered by density with less dense layers surrounding the progressively denser, inner ones, explains the structure of not only the Earth, but all of the planets. All we have to do is remember where all of these elements came from in the first place.
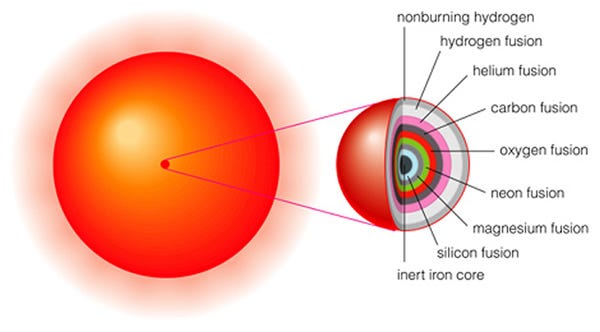
When the Universe was very young — only a few minutes old — practically the only elements that existed were hydrogen and helium. All the heavier ones were made in stars, and it was only when these stars died that these heavy elements were recycled back out into the Universe, allowing new generations of stars to form.
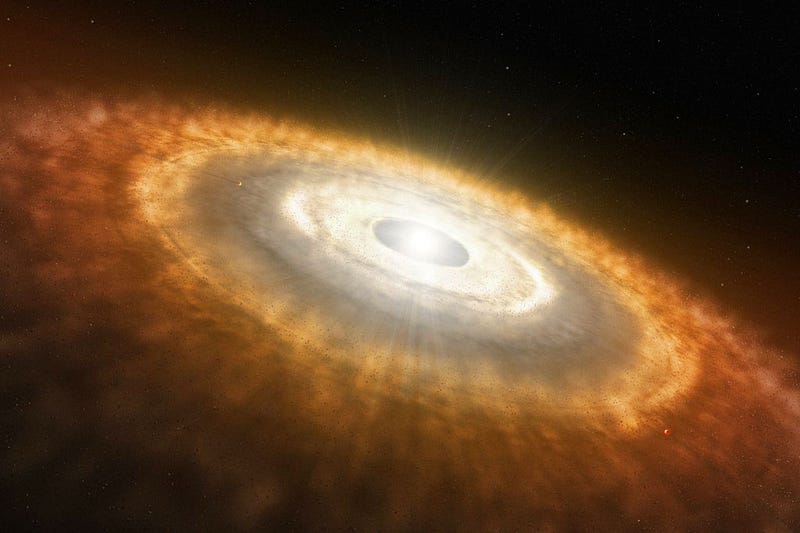
But this time, a mix of all of these new elements — not just hydrogen and helium, but carbon, nitrogen, oxygen, silicon, magnesium, sulphur, iron and more — goes into forming not only new stars, but a protoplanetary disk around each of those stars.
The outward pressure from the newly forming star preferentially pushes the lighter elements out towards the outer parts of the solar system, while gravity causes instabilities in the disk to collapse and form what will become planets.
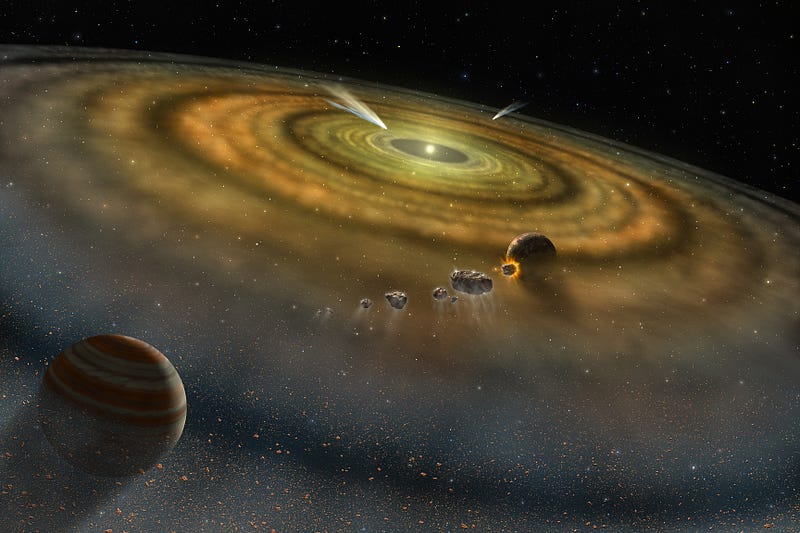
In the case of our Solar System, the four innermost worlds are the four densest planets in our Solar System, with Mercury being composed of the densest elements. All four of these were unable to gravitationally hold on to the large amounts of hydrogen and helium they formed with, preventing them from becoming gas giants like our Solar System’s other four planets.
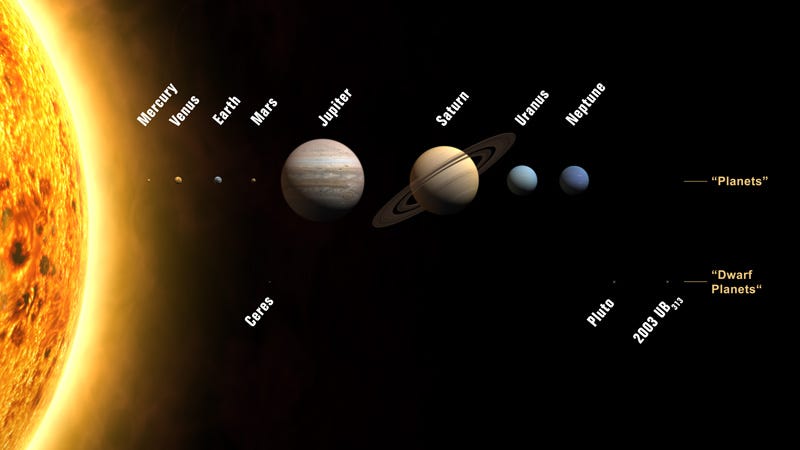
But the outer planets, being both more massive and farther away from the Sun (and hence receiving less radiation), managed to hang on to large amounts of these ultra-light elements, and formed gas giants.
Each of these worlds, much like the Earth, has — overall — the densest elements concentrated at the core, with lighter ones forming progressively less and less dense layers surrounding it.
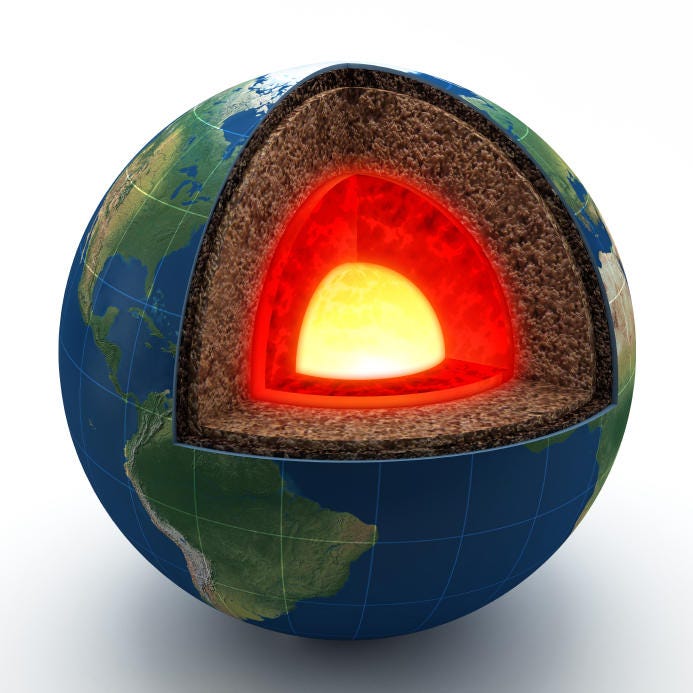
It should come as no great surprise that iron, the most stable element and the heaviest element made in great abundance outside of supernovae, is the most abundant element in the Earth’s core. But it may surprise you to learn that, in between the solid inner core and the solid mantle, lies a liquid layer more than 2,000 kilometers thick: the Earth’s outer core.
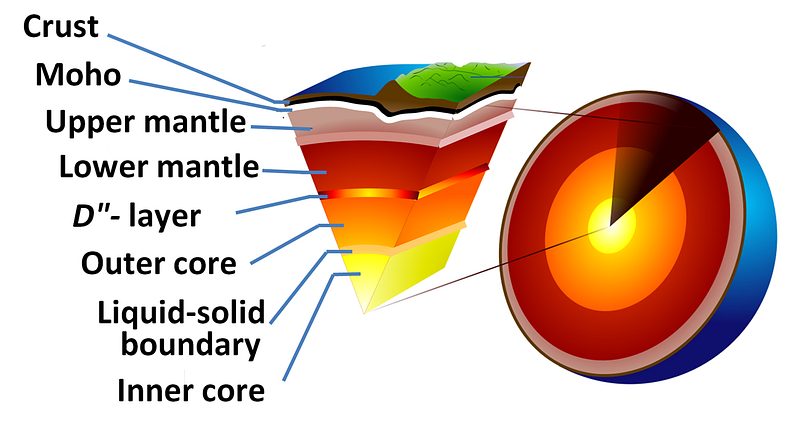
Much like the disgusting gum your grandma used to carry around, the Earth has a huge liquid layer inside of it, containing a full 30 percent of our planet’s mass! The way we know that the outer core is liquid is quite brilliant: from the seismic waves produced from earthquakes!
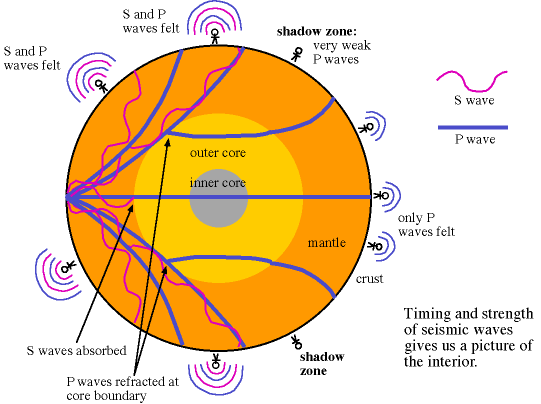
There are two different types of seismic waves produced in earthquakes: the primary compression wave, known as the P-wave, which works like a pulse through a slinky,
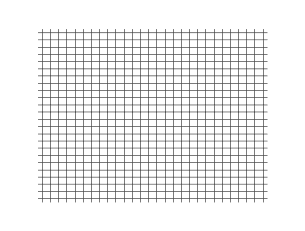
and the secondary shear wave, known as the S-Wave, which propagates like waves on the surface of the sea.
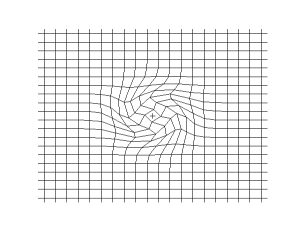
Both waves travel in a spherical shell outward from their point of origin on the Earth, striking and rippling through not only the surface near their epicenter, but all over the world! Seismic monitoring stations across the globe are equipped to pick up both P- and S-waves, but S-waves do not travel through liquid (they are attenuated, though), while P-waves not only do travel through liquid, they are refracted!
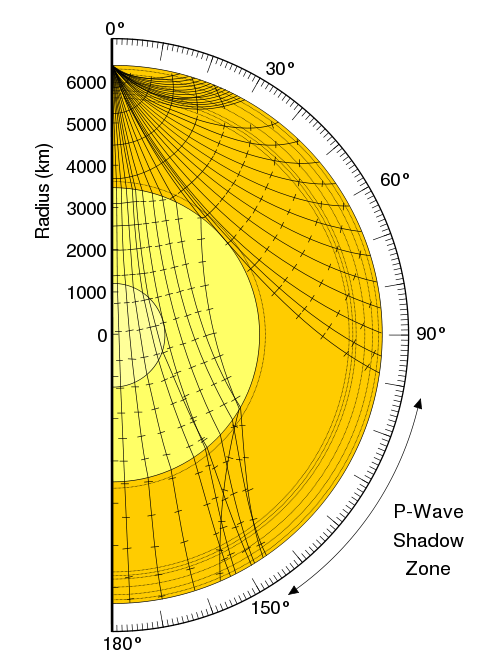
As a result of this, we can learn that the Earth has a liquid outer core, a solid mantle exterior to that, and a solid core interior to it! So that’s how come the Earth has the heaviest, densest elements at its core, and how we know its outer core is a liquid layer.
But why is the outer core liquid? Like all elements, whether iron is solid, liquid, gas or “other” depends on both the pressure and temperature of the iron.
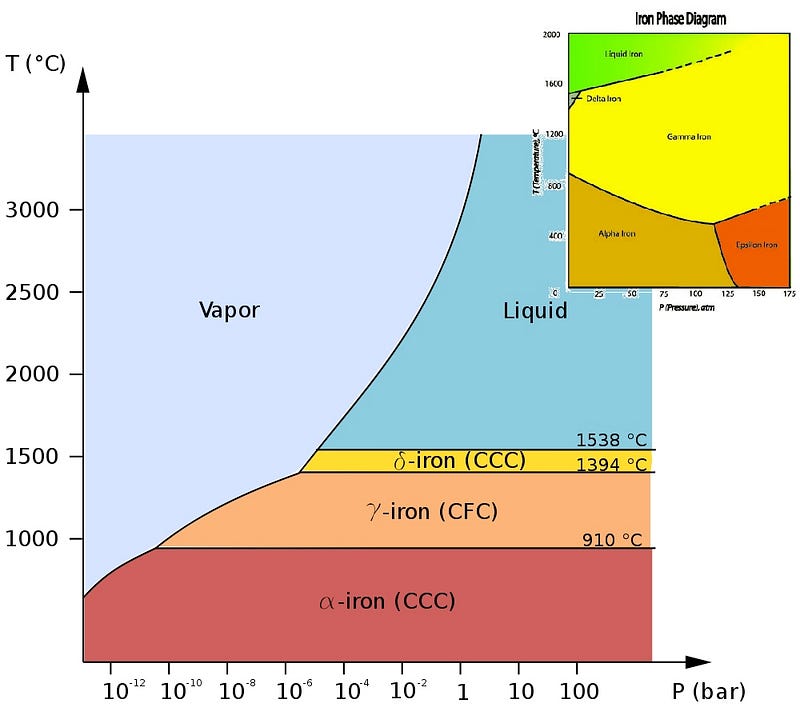
Iron, however, is much more complicated than many elements you may be used to. Sure, it can take on a variety of crystalline solid phases, as shown above, but we’re not interested in these normal pressures, shown in the diagrams above. We’re going all the way down into the core of the Earth, where the pressure isn’t just a few times (or even a few hundred times) the atmospheric pressure we’re used to, but rather millions of times what it is at sea level. What does the phase diagram look like for excessive pressures like that?
The wonderful thing about science is that even when you don’t know the answer off the top of your head, chances are, someone’s done the research where you can find the answer! In this case, Ahrens, Collins and Chen, 2001 have the answer we’re looking for!
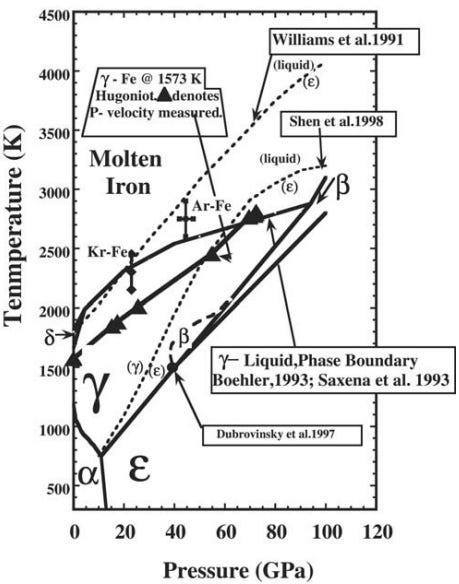
While this diagram shows tremendous pressures — up to 120 GigaPascals — it’s important to remember that our atmosphere has only 0.0001 GigaPascals, while the inner core experiences pressures of an estimated 330-360 Gpa! The topmost solid line represents the boundary between molten iron (above the line) and solid iron (below it). But notice how, right at the very edge of the solid line, it takes a sharp upwards turn?
At 330 GigaPascals, it takes a tremendous temperature, something comparable to those found at the surface of the Sun, to melt iron. Those same temperatures, however, at lower pressures, will easily keep iron in its liquid phase, while at higher pressures will see iron form a solid. What does this mean for the core of the Earth?
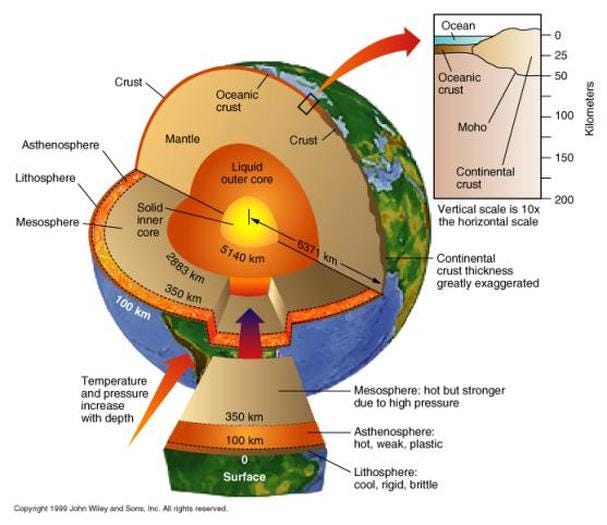
The highest temperature — at the center of the Earth — that our planet achieves is a little under 6,000 Kelvin, while the melting temperature of iron at the inner core/outer core boundary is most recently estimated to be right around that value as well.
But here’s the kicker: the Earth cools over time, as its heat is radiated into outer space faster than it generates its own heat from radioactive decay. Inside the Earth, its interior temperature drops, while its pressure remains constant.
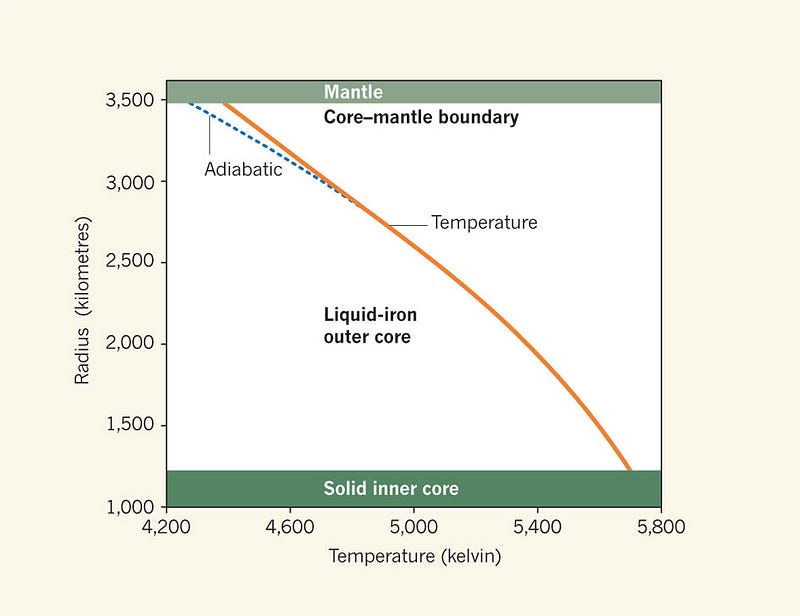
In other words, when the Earth first formed, it was hotter; it’s very likely that the entire core was once liquid, and as it continues to cool, the inner core continues to grow! And as this happens, because solid iron has a higher density than liquid iron, the Earth will contract slightly, necessitating what?
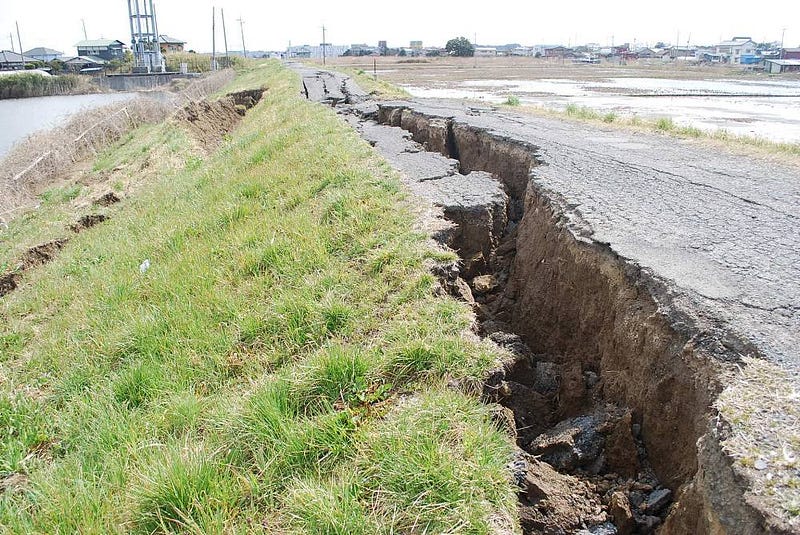
More earthquakes!
So the Earth’s core is liquid because it’s hot enough to melt iron, but only in places where the pressure is low enough. As the Earth continues to age and cool, more and more of the core becomes solid, and when it does, the Earth shrinks a little bit!
If we want to look far into the future, we can expect to eventually acquire features like the great scarps found on Mercury!
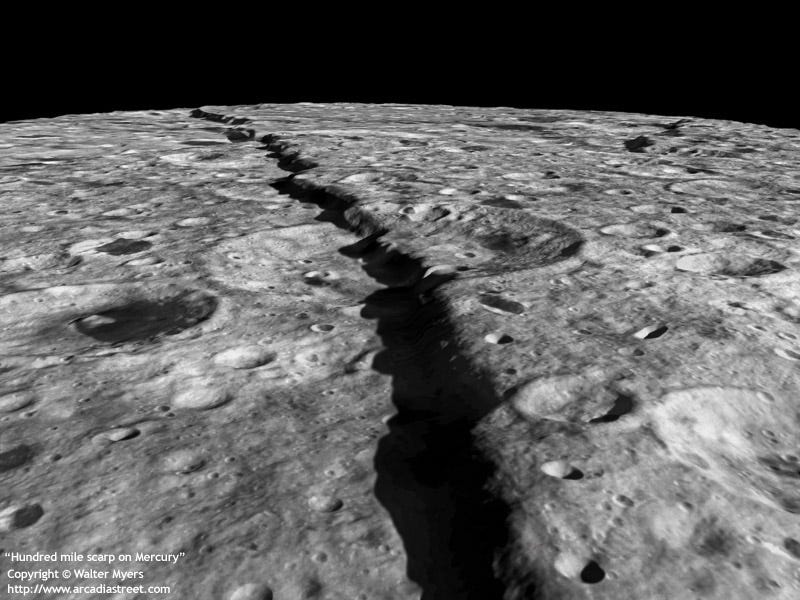
Because it’s so small, Mercury has already cooled and contracted a tremendous amount, and has hundred-mile-long cracks in it from where it was forced to contract due to this cooling!
So, ultimately, why does the Earth have a liquid core? Because it hasn’t finished cooling yet! And every earthquake you feel is the Earth getting just a little bit closer to its final, cooled-off, solid-all-the-way-through state!
(Don’t worry, though, the Sun will explode and you and everyone you know will be dead for a really long time before that ever happens!)
An earlier version of this post originally appeared on the old Starts With A Bang blog at Scienceblogs.