We Have No Idea What Makes A Planet ‘Potentially Habitable’
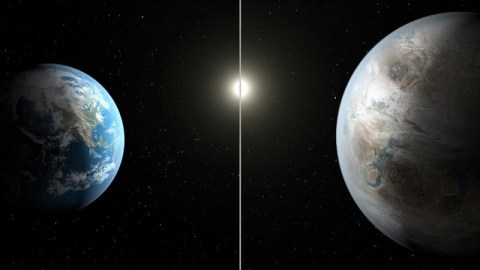
How many potentially habitable planets are there? We sincerely don’t know.
One of the most compelling scientific goals humanity has set for itself is to find extraterrestrial life: biological activity originating and continuing to occur on a world beyond Earth. It isn’t just our imaginations that have run wild with this possibility, it’s that we have a lot of indirect evidence identifying other potential locations where life could have arisen through similar processes to whatever occurred on Earth in our past. If we compare what’s out there with our expectations for what life requires, there’s a lot that appears to make sense.
While it might be a fun exercise to speculate about how many “potentially habitable” planets there might be out there — in our Solar System, in the Milky Way, in the Local Group, or even in the entire observable Universe — we have to be up front and honest about the assumptions that go into those estimates. These assumptions are all reflections of our ignorance, and the most uncomfortable fact of all cannot be ignored: in all the Universe, the only place we know of for certain where life has arisen is our own planet. Everything else is speculation. If we’re being completely honest with ourselves, we must admit we have no idea what makes a planet “potentially habitable.”
If we didn’t know anything else about the Universe other than the facts that we live on planet Earth and that life exists here, we would still have every reason to speculate about what else might be out there. After all:
- we live on a world that formed naturally,
- made of raw ingredients — atoms, molecules, etc. — that formed naturally,
- around a star that’s outputted energy at a relatively stable rate over billions of years,
- and life on our planet formed, at the latest, only a few hundred million years after the Earth itself formed.
If there was a natural explanation for how life on our world arose, and it’s eminently reasonable to assume that’s the case, then if other worlds have conditions on them that are similarly life-friendly to whatever we had on Earth in its early days, then perhaps those worlds could have had life arise on them, too. As long as the rules governing the Universe are the same everywhere, then all we need to do is discover and identify the worlds where the same processes that occurred to create life on Earth, and perhaps investigating those “potentially habitable” worlds will reveal life there as well.
Of course, that’s easier said than done. Why’s that? Because we run into our first great unknown: we don’t know how life was first created. Even if we look at the full body of scientific knowledge we have today, there’s a gap in the most important spot. We know how stars form, how solar systems form, and how planets form. We know how atomic nuclei form, how they fuse together in the interiors of stars to form heavy elements, and how those elements are recycled into the Universe to participate in complex chemistry.
And we know how chemistry works: atoms binding together to produce molecules in a wide variety of configurations, naturally. We find those complex molecules all over the Universe, from the interiors of meteorites to the ejecta of young stars to interstellar gas clouds to the protoplanetary disks in the process of creating planets.
But even with all of this, we don’t know how to go from complex, inorganic chemistry to a bona fide biological organism. Put simply, we don’t know how to create life from non-life.
It’s not being hyperbolic, either, to say “we don’t know” in this situation. Despite:
- searches to the limits of our ability for biological activity on other planets in our solar system,
- spectroscopic imaging of the atmospheres of every exoplanet atmosphere we can obtain spectra from,
- direct imaging of a variety of exoplanets involving a decomposition of their light,
- attempts to synthesize life from non-life in laboratory settings,
- and searches for technosignatures from potentially intelligent civilizations anywhere we’re capable of searching,
we have absolutely no evidence that favors life’s existence on any known world other than Earth. Despite all the suggestive evidence we’ve gathered that support the possibility of life arising in a myriad of different places, we’ve only ever found compelling evidence of it in two places: Earth, and on places we’ve sent Earth-based life to.
That isn’t to say that we know nothing about the possibility for life elsewhere. We know a lot, and we’re continuing to learn more with each new piece of information we gather. We know, for example, how to measure, count, and categorize stars in our own neighborhood, throughout the galaxy, and even throughout the Universe. We’ve learned that Sun-like stars are common, with about 15–20% of stars having comparable temperatures, luminosities, and lifetimes to our Sun.
About 75–80% of stars, interestingly enough, are red dwarfs: lower in temperature, lower in luminosity, and much longer-lived than our Sun. Although there are many important ways these systems are different from our own — planetary orbits are shorter; their planets should be tidally locked; they flare frequently; these stars emit disproportionate amounts of ionizing radiation — we don’t have any way of assessing whether planets around these stars are similarly habitable (much less more habitable) to planets around stars like our Sun. In the absence of evidence, we can draw no robust conclusions.
What about the lessons we’ve learned from our own Solar System? Earth may be unique among the worlds we have right here in our cosmic backyard as being the only planet to be obviously covered with life, but we may not be the only world that either had life in its past or that may have life persisting on it today.
Mars likely had liquid water on its surface for over a billion years before it froze; could life have thrived there in our Solar System’s ancient history? And could that life survive in a sub-surface reservoir today?
Venus may have had a more temperate past with liquid water on its surface for quite some time. Could it have given rise to life, and could that life persist in the Venusian cloud-decks or cloud-tops, where conditions are far more similar to Earth?
What about sub-surface oceans with tidal heating, present on ice-covered worlds like Enceladus, Europa, Triton, or Pluto? What about life on worlds with liquid methane rather than liquid water, such as Titan? What about large worlds with potential groundwater, such as Ganymede?
Until we’ve exhaustively investigated these nearby worlds, we must admit our ignorance: we don’t even know how inhabited our Solar System is.
What about life persisting in or even arising from interstellar space? Although this idea may sound far-fetched to many, if we trace back the history of life on Earth, it appears to be quite complex — with tens of thousands of base pairs of nucleic acids encoding information — from the moment it first arose.
Meanwhile, if we look back at the raw ingredients that we find throughout the Universe, they’re not just simple, inert molecules. We find organic molecules like sugars, amino acids, and ethyl formate: the molecule that gives raspberries their scent. We find complex carbon-based molecules, like polycyclic aromatic hydrocarbons.
We even find more amino acids that occur naturally than are involved in life processes on Earth. While we only have 20 active amino acids, all of which have the same “handedness” or chirality, the Murchison meteorite alone has some ~80+ unique amino acids in it, some of which are left-handed and others of which are right-handed. Despite the success we’ve had on Earth, we simply don’t know whether other pathways are not only possible for life, but potentially even more likely.
What about our environment? Would a star system with greater percentages of heavy elements (or smaller percentages) have a greater chance at life arising and thriving than ours does? What about having a gas giant like Jupiter out near the frost line; is that beneficial, benign, or actually harmful? What about our position within the galaxy; is that special or mundane? Of the ~400 billion stars in our galaxy, we don’t even know what criteria to look for when trying to select what targets might be good candidates for life.
And yet, you can find statements made all the time akin to the one that went viral just a few weeks ago: that there are 300 million potentially habitable planets right here in the Milky Way galaxy. They’ve been made before, and they’ll be made again plenty of times before we actually have our next meaningful data point: a world beyond Earth where we’ve found a compelling, robust biosignature (or at least a bio-hint). Until that day comes, you should treat all of those headlines with extreme skepticism, as we know too little about the habitability of planets to even discuss what it means to be “potentially habitable.”
This isn’t to diminish the tremendous advances we’re actually making in the field of exoplanet sciences. Thanks to the combination of ultra-sensitive telescopes to periodic changes in a star’s brightness like NASA’s Kepler and TESS with large ground-based telescopes that can measure the periodic shifts in the star’s spectral lines, we’ve revealed thousands of confirmed planets around other stars. In particular, where the data is best, we can measure:
- the mass, radius, and temperature of the star,
- the mass, radius, and orbital period of the planet,
and that allows us to infer what the surface temperature of that planet should be, assuming it has an atmosphere similar to Earth’s. Now, all of that might sound reasonable, and it might sound reasonable to equate “potentially habitable” with “and it has the right temperatures so that liquid water could survive on its surface,” but that’s predicated on a lot of assumptions that are supported by only flimsy evidence. The truth is that we need superior data before we can draw any meaningful conclusions about habitability.
In the quest for life beyond Earth, it’s important to remain both honest in where we are today and open-minded for what we might find in the future. We know that life arose (or arrived) on Earth very early, and survived and thrived ever since. We know that if we’re looking for planets with similar histories, properties, and conditions, we’re likely to find any nearby planets that may have had similar successes. That’s the conservative way to look, and it’s eminently sensible.
But thinking only along these lines could be existentially limiting. We don’t know whether other, very different worlds with very different histories, properties, and conditions might be as likely or even more likely to have life on them than Earth was. We don’t know how those probabilities are distributed across the myriad of planets present in our Universe. And we don’t know what the odds of developing complex, differentiated, macroscopic, or even intelligent life are if the early seeds of life do take hold. We have every reason to believe that life exists elsewhere in the Universe, and every motivation to go looking for it. But until we have an better idea of what is and isn’t inhabited, we have no business asserting how many “potentially habitable” worlds there might actually be.
Starts With A Bang is written by Ethan Siegel, Ph.D., author of Beyond The Galaxy, and Treknology: The Science of Star Trek from Tricorders to Warp Drive.