There’s Almost No Antimatter In The Universe, And No One Knows Why
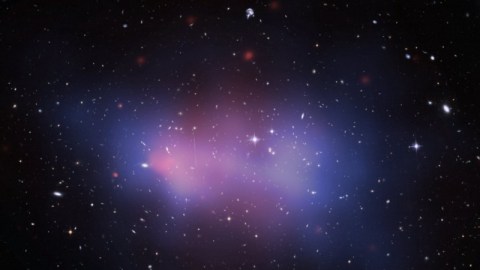
The Universe is filled with something, as opposed to nothing, and scientists don’t understand it.
When we look around at the Universe:
- at the planets and stars,
- at the galaxies and clusters of galaxies,
- and at the gas, dust and plasma populating the space between these dense structures,
we find the same signatures everywhere. We see atomic absorption and emission lines, we see matter interacting with other forms of matter, we see star formation and stellar death, collisions, X-rays and so much more. There’s an obvious question that cries out for an explanation: why is there all this stuff, rather than nothing at all? If the laws of physics are symmetric between matter and antimatter, the Universe we see today should be impossible. Yet here we are, and no one knows why.
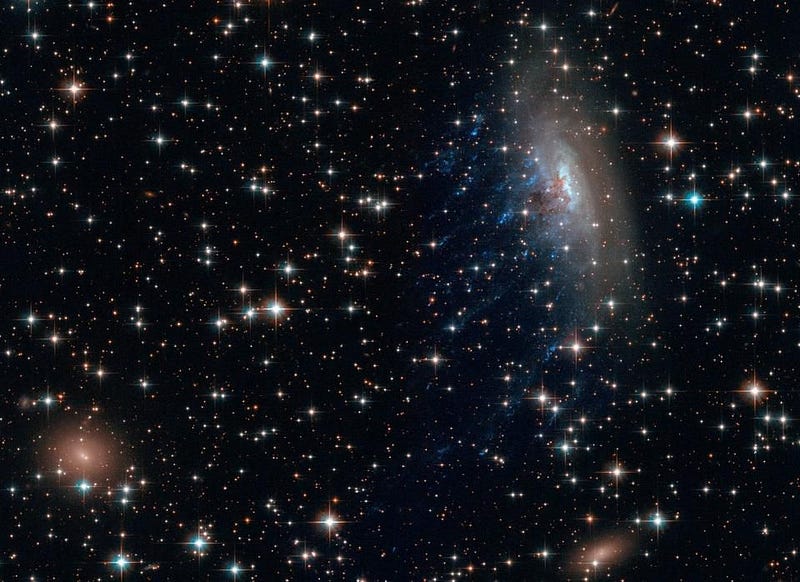
Think about these two seemingly contradictory facts:
1.) Every interaction between particles that we’ve ever observed, at all energies, has never created or destroyed a single particle of matter without also creating or destroying an equal number of antimatter particles. The physical symmetry between matter and antimatter is even more stringent than this:
- every time we create a quark or lepton, we also create an antiquark or antilepton,
- every time a quark or lepton is destroyed, an antiquark or antilepton is also destroyed,
- the created or destroyed leptons and antileptons must balance across each lepton family, and
- every time a quark or lepton experiences an interaction, collision or decay, the total net number of quarks and leptons at the end of the reaction (quarks minus antiquarks, leptons minus antileptons) is the same at the end as it was at the beginning.
The only way we’ve ever changed the amount of matter in the Universe has been to also change the Universe’s antimatter by an equal amount.
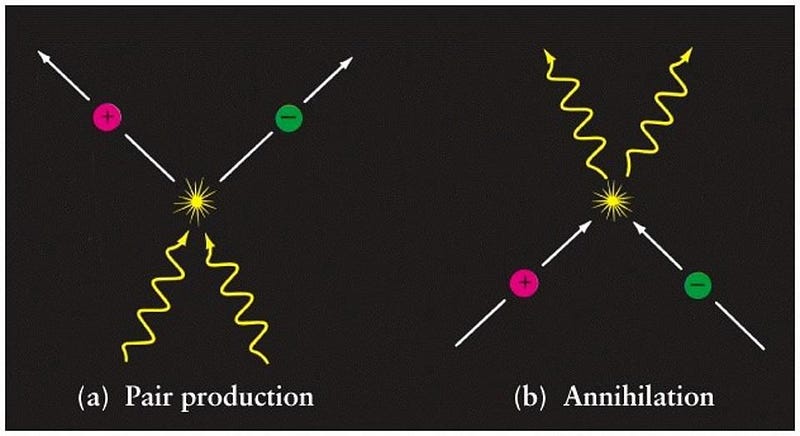
And yet, there’s this second fact:
2.) When we look out at the Universe, at all the stars, galaxies, gas clouds, clusters, superclusters and largest-scale structures everywhere, everything appears to be made of matter and not antimatter. Whenever and wherever antimatter and matter meet in the Universe, there’s a fantastic outburst of energy due to particle-antiparticle annihilation.
But we don’t see any signatures of matter annihilating with antimatter on the largest scales. We don’t see any evidence that some of the stars, galaxies or planets we’ve observed are made of antimatter. We don’t see the characteristic gamma rays that we’d expect to see if some antimatter parts were colliding (and annihilating) with the matter parts. Instead, it’s matter, matter everywhere, in the same abundance everywhere we look.
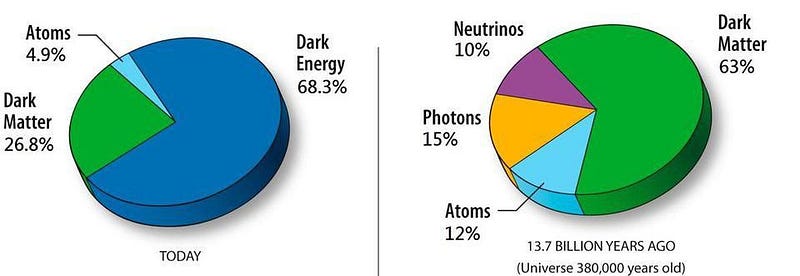
It seems like an impossibility. On one hand, there is no known way, given the particles and their interactions in the Universe, to make more matter than antimatter. On the other hand, everything we see is definitely made of matter and not antimatter.
We’ve actually observed matter-antimatter annihilation in some extreme astrophysical environments, but only around hyper-energetic sources that produce matter and antimatter in equal amounts, such as massive black holes. When the antimatter runs into matter in the Universe, it produces gamma rays of very specific frequencies, which we can then detect. The interstellar and intergalactic medium is full of material, and the complete lack of these gamma rays is a strong signal that there aren’t large amounts of antimatter particles flying around anywhere, since that matter/antimatter signature would show up.
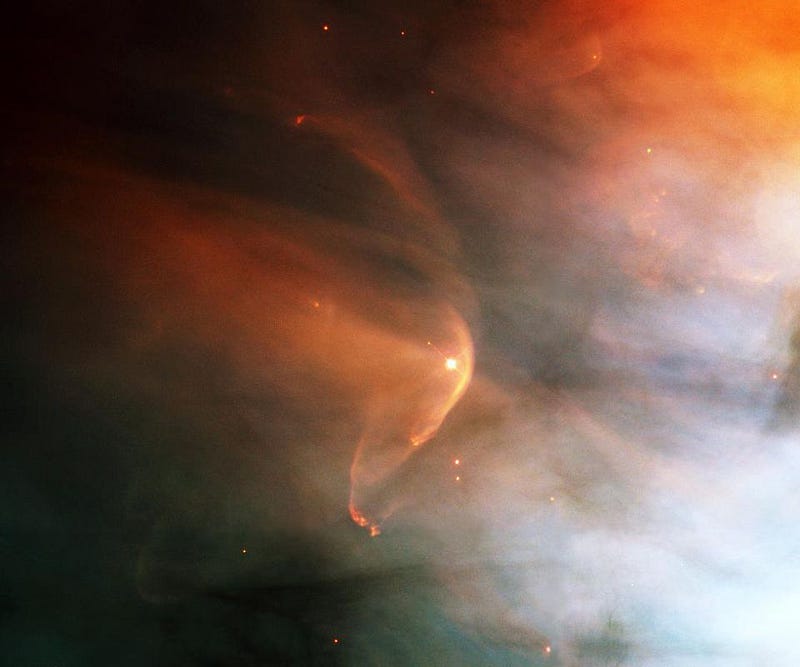
If you threw a single antimatter particle into the mix of our galaxy, it would only last for about 300 years before annihilating with a matter particle. That constraint tells us, within the Milky Way, the amount of antimatter can be no more than 1 part in a quadrilliion (10¹⁵) compared to the total amount of matter.
On larger scales — of satellite galaxies, major, Milky Way-scale galaxies and even the scales of galaxy clusters — the constraints are less stringent but still very strong. With observations spanning distances ranging from a few million light-years away to over three billion light-years distant, we’ve observed a dearth of the X-rays and gamma rays we’d expect from matter-antimatter annihilation. Even on large, cosmological scales, 99.999%+ of what exists in our Universe is definitely matter (like us) and not antimatter.
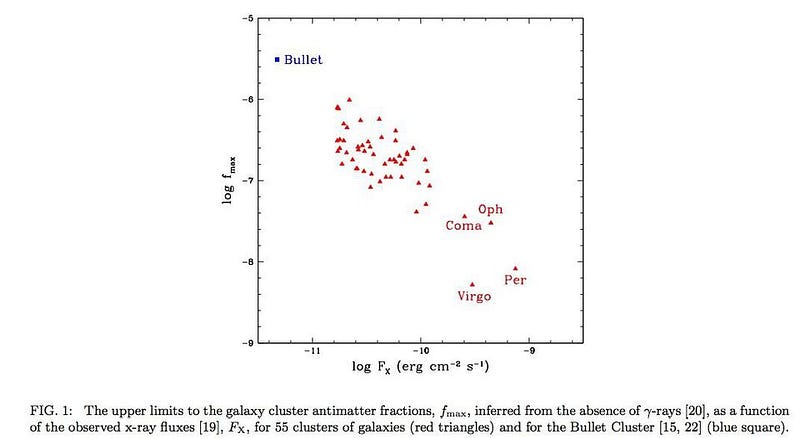
So how did we get here today, with a Universe made of a lot of matter and practically no antimatter, if the laws of nature are completely symmetric between matter and antimatter? Well, there are two options: either the Universe was born with more matter than antimatter, or something happened early on, when the Universe was very hot and dense, to create a matter/antimatter asymmetry where there was none initially.
That first idea is scientifically untestable without recreating the entire Universe, but the second one is quite compelling. If our Universe somehow created a matter/antimatter asymmetry where there initially wasn’t one, then the rules that were at play back then should remain unchanged today. If we’re clever enough, we can devise experimental tests to uncover the origin of the matter in our Universe.
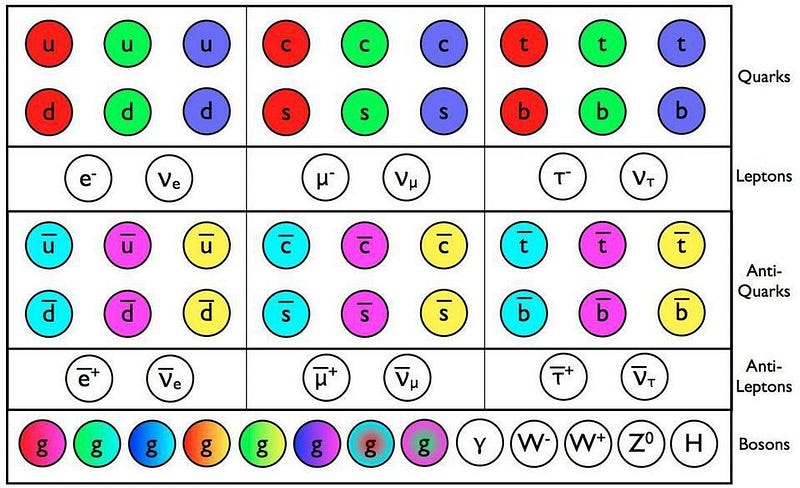
In the late 1960s, physicist Andrei Sakharov identified three conditions necessary for baryogenesis, or the creation of more baryons (protons and neutrons) than anti-baryons. They are as follows:
- The Universe must be an out-of-equilibrium system.
- It must exhibit C– and CP-violation.
- There must be baryon-number-violating interactions.
The first one is easy, because an expanding, cooling Universe with unstable particles (and/or antiparticles) in it is, by definition, out of equilibrium. The second one is easy, too, since “C” symmetry (replacing particles with antiparticles) and “CP” symmetry (replacing particles with mirror-reflected antiparticles) are both violated in many weak interactions involving strange, charm, and bottom quarks.
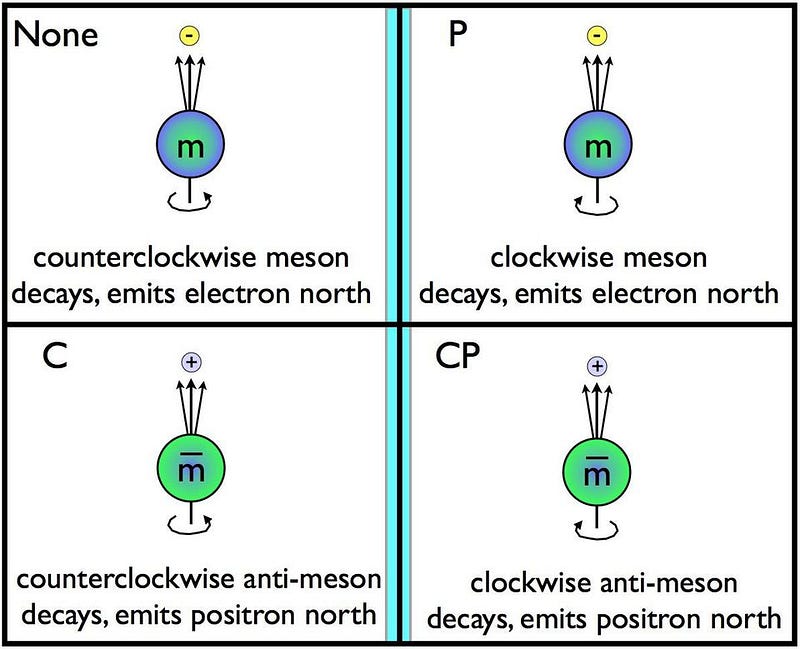
That leaves the question of how to violate baryon number. Experimentally, we’ve seen that the balance of quarks to antiquarks and leptons to antileptons are each explicitly conserved. But in the Standard Model of particle physics, there isn’t an explicit conservation law for either one of those quantities individually.
It takes three quarks to make a baryon, so for every three quarks we assign a baryon number (B) of 1. Similarly, every lepton has a lepton number (L) of 1. Antiquarks, antibaryons, and antileptons all have negative B and L numbers, correspondingly.
But according to the Standard Model, it’s only the difference between baryons and leptons, B — L, that’s conserved. Under the right circumstances, you could not only make extra protons, you can make the electrons you need to go with them. Those exact circumstances may be unknown, but the hot Big Bang gave them an opportunity to arise.
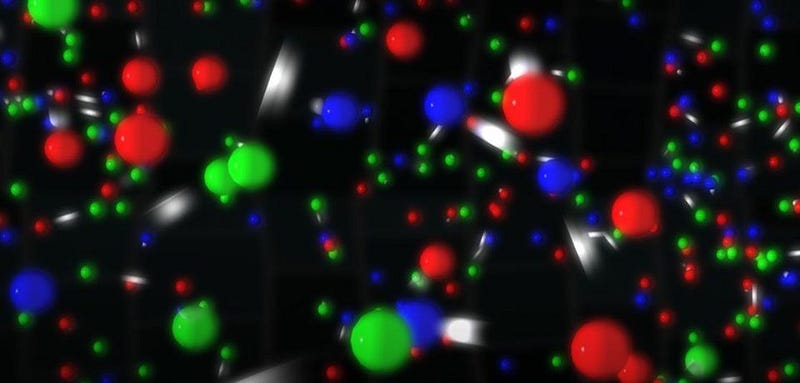
The earliest stages of the Universe are described by incredibly high energies: high enough to create every known particle and antiparticle in great abundance via Einstein’s famous E = mc². If particle creation and annihilation works the way we think it does, the early Universe should be filled with equal amounts of matter and antimatter particles, all interconverting into one another as the available energy remains extremely high.
As the Universe expands and cools, unstable particles, once created in great abundance, will decay. If the right conditions are met — specifically, the three Sakharov conditions — they can lead to an excess of matter over antimatter, even where there was none initially. The challenge for physicists is generating a viable scenario, consistent with observations and experiments, that can give you enough of an excess of matter over antimatter.
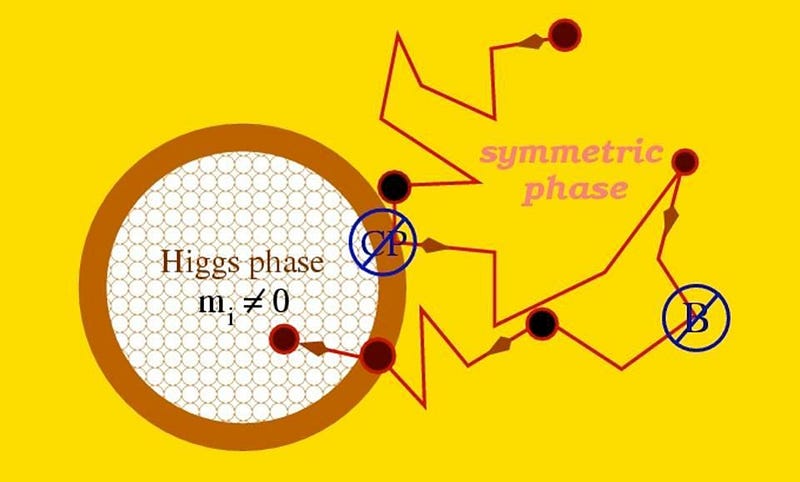
There are three leading possibilities for how this excess of matter over antimatter could have emerged:
- New physics at the electroweak scale could greatly enhances the amount of C– and CP-violation in the Universe, leading to an asymmetry between matter and antimatter. Standard Model interactions (through the sphaleron process), which violate B and L individually (but still conserve B — L) can then generate the right amounts of baryons and leptons.
- New neutrino physics at high energies, of which we have a tremendous hint, could create a fundamental lepton asymmetry early on: leptogenesis. The sphalerons, which conserve B — L, could then use that lepton asymmetry to generate a baryon asymmetry.
- Or GUT-scale baryogenesis, where new physics (and new particles) are found to exist at the grand unification scale, where the electroweak force unifies with the strong force.
These scenarios all have some elements in common, so let’s walk through the last one, just as an example, to see what could have happened.
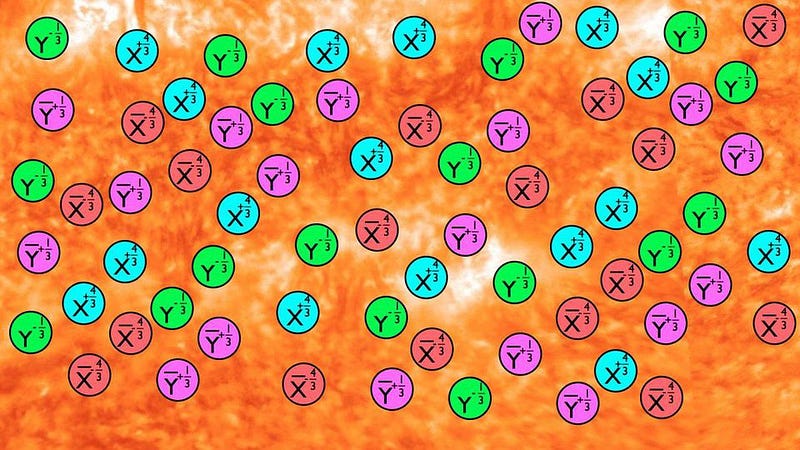
If grand unification is true, then there ought to be new, super-heavy particles, called X and Y, which have both baryon-like and lepton-like properties. There also ought to be their antimatter counterparts: anti-X and anti-Y, with the opposite B — L numbers and the opposite charges, but the same mass and lifetime. These particle-antiparticle pairs can be created in great abundance at high enough energies, and then will decay at later times.
So your Universe can be filled with them, and then they’ll decay. If you have C– and CP-violation, however, then it’s possible that there are slight differences between how the particles and antiparticles (X/Y vs. anti-X/anti-Y) decay.
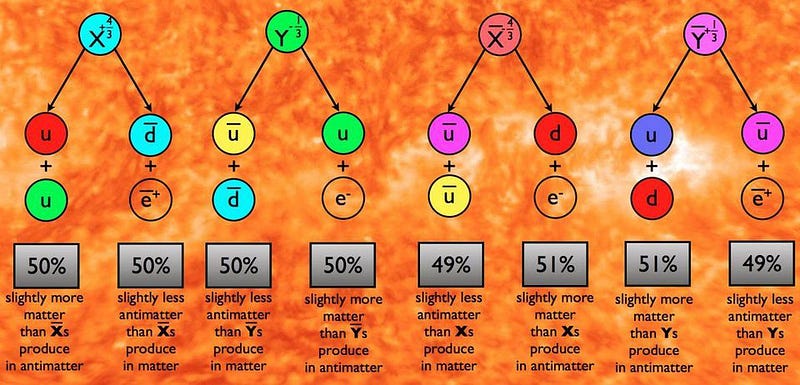
If your X-particle has two pathways: decaying into two up quarks or an anti-down quark and a positron, then the anti-X has to have two corresponding pathways: two anti-up quarks or a down quark and an electron. Notice that the X has B — L of two-thirds in both cases, while the anti-X has negative two-thirds. It’s similar for the Y/anti-Yparticles. But there is one important difference that’s allowed with C– and CP-violation: the X could be more likely to decay into two up quarks than the anti-X is to decay into two anti-up quarks, while the anti-X could be more likely to decay into a down quark and an electron than the X is to decay into an anti-down quark and a positron.
If you have enough X/anti-X and Y/anti-Y pairs, and they decay in this allowed fashion, you can easily make an excess of baryons over antibaryons (and leptons over anti-leptons) where there was none previously.
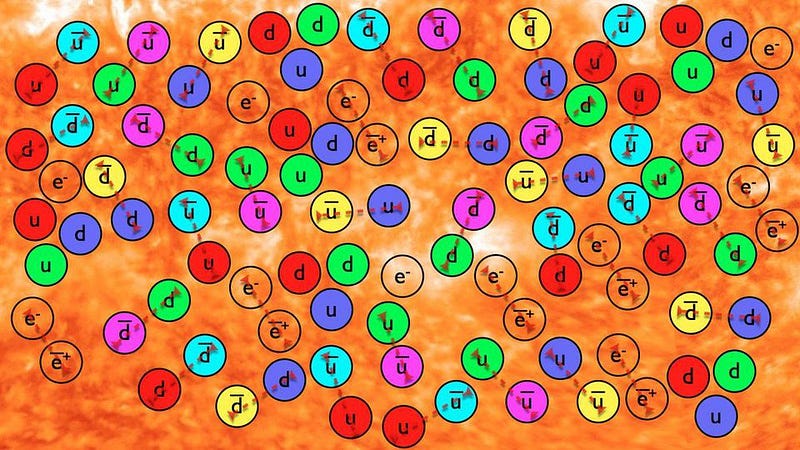
That’s one example illustrating how we think it must have happened. We started with a completely symmetric Universe, obeying all the known laws of physics and beginning with a hot, dense, rich state full of both matter and antimatter in equal amounts. Through some yet-to-be-determined mechanism, one that obeys the three Sakharov conditions, these natural processes generated an excess of matter over antimatter in the end.
The fact that we exist and are made of matter is indisputable; the question of why our Universe contains something (matter) instead of nothing (from an equal mix of matter and antimatter annihilating away) is still an unanswered one . This century, advances in precision electroweak testing, collider technology, neutrino physics, and experiments probing beyond the Standard Model have a chance to reveal exactly how it happened. Until then, we can be certain that there’s almost no antimatter in the Universe, but no one knows why.
Ethan Siegel is the author of Beyond the Galaxy and Treknology. You can pre-order his third book, currently in development: the Encyclopaedia Cosmologica.