Missing Matter Found, But Doesn’t Dent Dark Matter
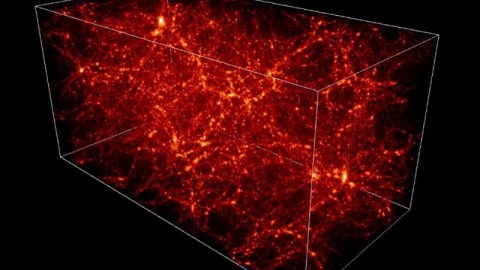
Finding a warm-hot intergalactic plasma is amazing! But we still need dark matter just as much as ever.
“There are stars leaving the Milky Way, and immense gas clouds falling into it. There are turbulent plasmas writhing with X- and gamma-rays and mighty stellar explosions. There are, perhaps, places which are outside our universe. The universe is vast and awesome, and for the first time we are becoming a part of it.” –Carl Sagan
Look out at the Universe as deeply as possible, and everywhere you look, there they are: stars and galaxies, beautiful, distant, and in all directions. All told, there are some two trillion galaxies in the observable Universe, each one with hundreds of billions of stars, on average. But if we take all that light, even knowing how stars work, it only explains a tiny fraction of the Universe’s mass. Looking within the galaxies themselves for gas, dust, black holes, nebulae, and more, we still don’t get close to enough mass to make up our Universe. A recent new set of studies have revealed new “missing matter” in between the galaxies for the first time, inching us closer. But even so, over 80% is completely unknown. Until we find dark matter, this mystery won’t be solved.
We know how much total matter there has to be in the Universe. The expansion rate is dependent on what’s present in the Universe, so measuring the Hubble flow of variable stars, galaxies, supernovae, etc., tells us how much matter, radiation, and other forms of energy need to be present. We can also measure the large-scale structure of the Universe, and from the clustering of galaxies on a variety of scales, determine how much total matter, as well as how much is normal and how much is dark, there needs to be. And the fluctuations in the cosmic microwave background, the Big Bang’s leftover glow, tell us a whole lot about not just the total amount of matter necessary to give the Universe, but how much is normal matter and how much is dark matter.
Finally, looking at the light elements left over from the Big Bang offers a completely independent piece of data: the total amount of normal (i.e., atom-based) matter that must exist. From all the different lines of evidence, we see the same picture. The fact that about 5% of the Universe’s energy is in normal matter, 27% is dark matter, and the other 68% is dark energy has been known for nearly 20 years now, but it remains as puzzling as ever. For instance:
- We still don’t know what dark energy is, or what causes it.
- We know from a slew of observations that dark matter exists, and we know its generic properties, but we have yet to directly detect it or find the particle(s) responsible for it.
- And even the normal matter — the stuff made of protons, neutrons, and electrons — isn’t fully accounted for.
In fact, if we add up all the normal matter we know about, we’re still missing the majority of it.
There are two ways to measure the Universe that are completely independent of one another: through the light that objects emit or absorb, and through the gravitational effects of matter. The earlier methods described — the expansion of the Universe, the large-scale structure, and the cosmic microwave background — all use gravity to make their measurements. But light plays a major role, too. Stars shine because of the internal physics that causes nuclear reactions inside them, and so measuring the light coming from all of them tells you how much mass there is. Measure the absorption and emission of other wavelengths of light, and you can calculate how much mass there is in not only stars, but gas, dust, nebulae, and black holes. Go to high energies, and you’ll even be able to measure hot plasmas within galaxies. But we’re still missing more than half, perhaps even up to 90%, of the total normal matter. In other words, of that 5%, we’re missing most of it.
So where should the rest of it be? Not in galaxies at all, but between them. Dark matter should clump and cluster together in large-scale filaments, but so should normal matter. When the high-energy radiation from the first stars passes through intergalactic space, the dark matter and light completely ignore each other, but the normal matter is vulnerable. Neutral atoms formed when the Universe was a mere 380,000 years old; after hundreds of millions of years, the hot, ultraviolet light from those early stars hits those intergalactic atoms. When it does, those photons get absorbed, kicking the electrons out of their atoms entirely, and creating an intergalactic plasma: the warm-hot intergalactic medium (WHIM).
Up until now, the WHIM has been mostly theoretical, as our tools haven’t been good enough to measure it except in a few rare locations. The WHIM should be very low in density, located along dark matter filaments, and at very high temperatures: between 100,000 K and 10,000,000 K. For the first time, now, there’s a statistically significant signal that exceeds the 5σ statistical significance mark, thanks to research by two independent teams. One, led by Anna de Graaff, looked at the cosmic web; one, led by Hideki Tanimura looked at the space between luminous red galaxies. Both of them detected the WHIM to greater than 5σ significance, and both used the same method to do it: the Sunyaev-Zel’dovich effect.
What is the Sunyaev-Zel’dovich effect? Imagine you’re sending light uniformly, in all directions, throughout the Universe. As it travels, the expansion of the Universe stretches it, causing it to fall to lower wavelengths. But in some places, it will pass through a hot, ionized plasma. When photons pass through a plasma, there’s a slight effect due to the electromagnetic, wave nature of light: the photons gets shifted to slightly higher energies, due to both the temperature and the motion of the plasma.
It was way back in 1969 that the Sunyaev-Zel’dovich paper predicting this effect came out, The interaction of matter and radiation in a hot-model universe, but it would be decades before the effect was first detected. In fact, the paper was written almost entirely by Sunyaev, with Zel’dovich merely adding in how difficult the effect would be to detect. Nearly 50 years later, we’ve used it to detect the missing normal matter in the Universe.
But this doesn’t eliminate the need for dark matter; it doesn’t touch that undiscovered 27% of matter in the Universe, not in the slightest. It’s another piece of that 5% that we know is out there, that we’re struggling to put together. It’s just protons, neutrons, and electrons, existing in about six times the abundance within these filaments as compared to the cosmic average. The fact that this filamentary structure contains normal matter at all is further evidence for dark matter, since without it there’d be no gravitationally overdense regions to hold the extra normal matter in place. In this case, the WHIM traces the dark matter, further confirming what we know must be out there.
Yes, we’ve found some of the missing matter in the Universe, and that’s incredible! But the missing matter we found was part of the normal matter — part of the 5% of the Universe that includes us — and leaves all of the dark matter untouched. The latest discovery suggests something incredible: that the missing baryon problem might be solved by looking to the great cosmic web that gave rise to everything we see. But that remaining 27% of the Universe must still be out there, and we still don’t know what that is. We can see its effects, but no amount of missing normal matter is going to make a dent in the dark matter problem. We still need it, and no matter how much normal matter we find, even if we get all of it, we’ll still only be 1/6th of the way to understanding all of the matter in our Universe.
Ethan Siegel is the author of Beyond the Galaxy and Treknology. You can pre-order his third book, currently in development: the Encyclopaedia Cosmologica.