Happy Anniversary To The Test That Showed ‘God Does Play Dice’ With The Universe
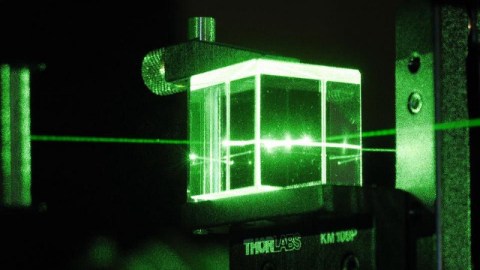
On September 27, 1972, scientists performed the first test of Bell’s inequality. God does play dice with the Universe, after all.
One of the most puzzling and counterintuitive aspects of quantum physics is the apparent link between determinism and measurement. Make a precise measurement of the quantum state of your particle — of its spin, its position, or which slit it went through — and you determine that property exactly. Choose not to make that measurement, and that property is indeterminate. To measure or not measure, mind-bogglingly, leads to different experimental outcomes.
Could this be right? Could there be a fundamental randomness to the Universe: an indeterminism that is inherent to nature itself? For generations, scientists argued whether the ability to predict only probabilities of outcomes meant quantum mechanics was incomplete. Was there more to nature than all we can see? Here’s the story of how, through cleverly-designed and carefully-executed experiments, we learned the answer.
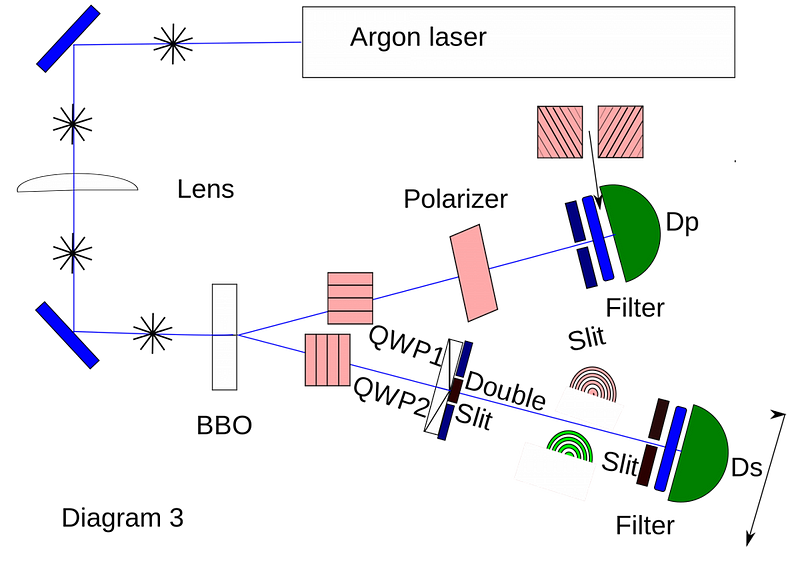
Quantum entanglement is the idea that you can create two quantum mechanical particles with linked but indeterminate properties. If you only have one of the particles, it will appear to behave exactly as a single quantum particle ought to behave: acting like a wave or particle as appropriate, with determined or indeterminate properties in accord with its measurement history. If you have both particles, however — or if you have one and another observer has the other — you’ll find that the results of one aren’t completely independent of the results of the other. Even if you take them an arbitrary distance apart and make measurements simultaneously (in any particular reference frame), the results will still display those quantum correlations.
This is what Einstein famously called, “spooky action-at-a-distance.”
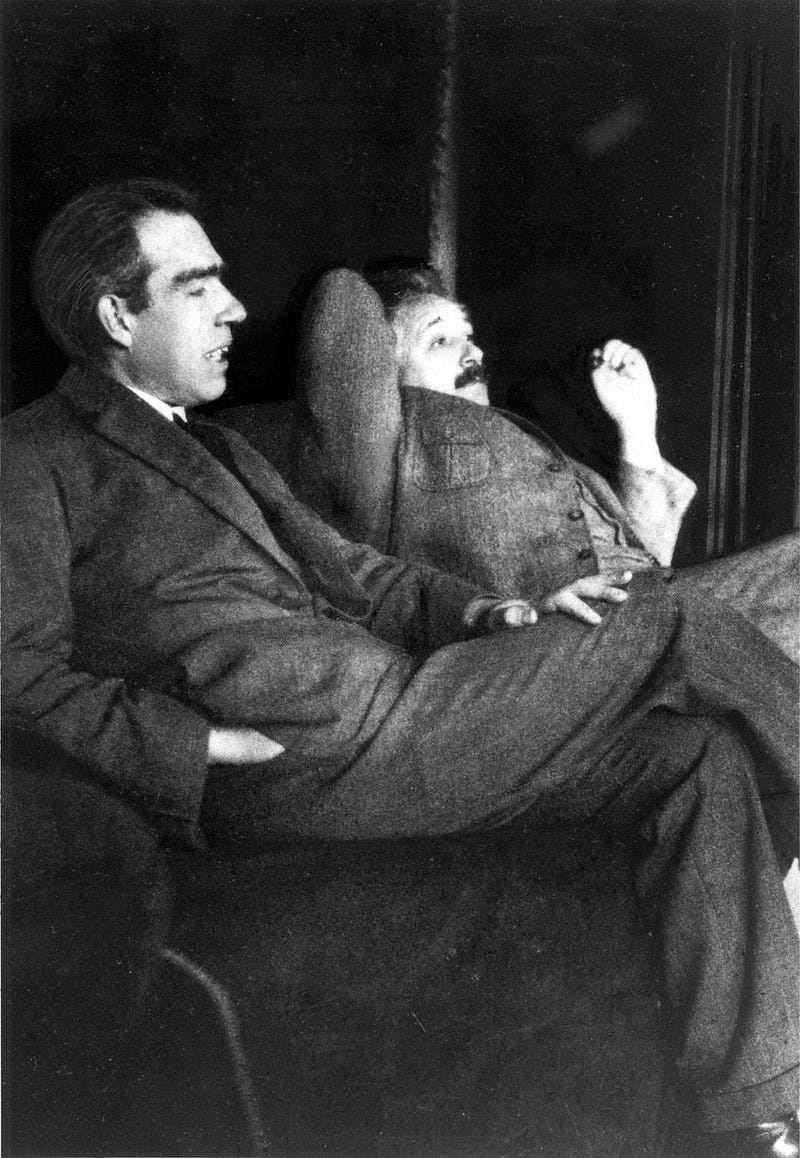
The spookiness is this: if you make a measurement of one particle, you determine its quantum state from an array of potential possibilities. But you also determine (or, at least, constrain) the outcome of the other particle, instantaneously, even if that particle is located somewhere distantly across the Universe.
If this sounds like it violates relativity to you, you’re not alone. This was the most troubling part about quantum physics to Einstein, the discoverer of relativity. Information isn’t transmitted from one particle to the other, but the correlations are still real. Even if you make these measurements faster than relativity would allow a signal to be transmitted, the effects of entanglement show up anyway.
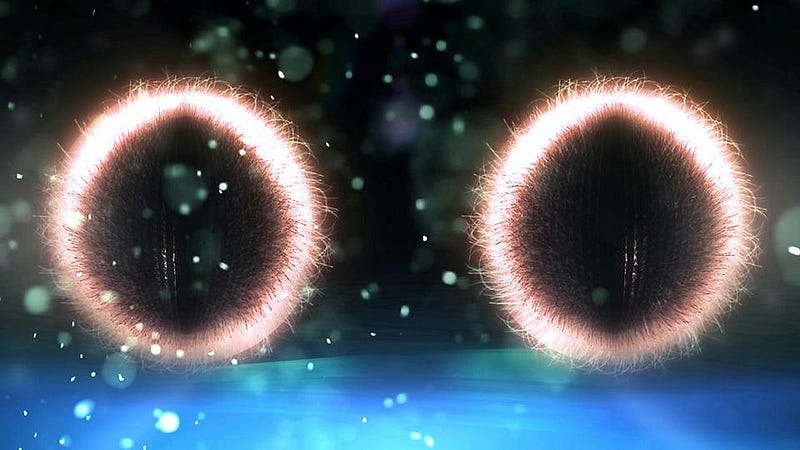
The big hope of Einstein (and others), in pointing out this puzzle, was that quantum mechanics might be shown to be incomplete. Perhaps, underlying reality, there were variables we could not see that determined these seemingly indeterminate quantum states, and this paradox would lead us there. Einstein, with his collaborators Boris Podolsky and Nathan Rosen, put out a scientific paper quantifying the poetic statement, “God does not play dice with the Universe.”
If there were hidden variables underlying reality, perhaps they could be the resolution to this conundrum. The key would be to devise an experiment capable of determining what the predictions of a hidden variable reality was, and if-and-how it was different from the standard quantum picture.
In 1964, physicist John Stewart Bell designed a thought experiment to address exactly this, using pairs of entangled particles. If there were hidden variables that determined reality, they would obey classical laws, rather than quantum laws. Bell was the first to quantify how the predictions of local realism and the predictions of quantum mechanics would be different, as shown by entangled pairs of particles.
Using one pair wouldn’t do; you’d have to take a large sample of pairs and statistically analyze them. But, for example, if you tried to measure the polarization spins (either + or -) of two entangled photons, the predictions for local realism and quantum mechanics are both dependent on the angle that a photon polarizer is oriented at. But their dependencies are slightly different from one another.
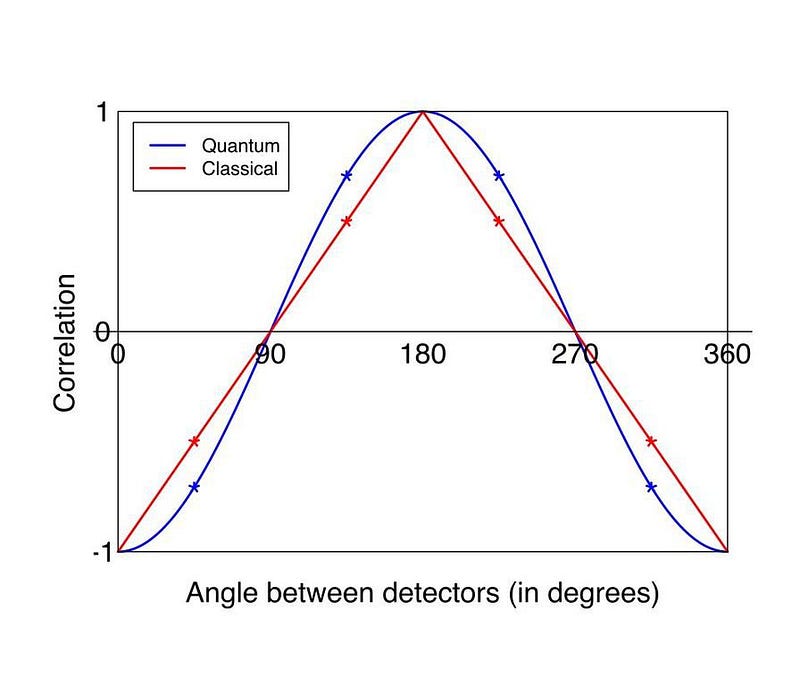
The first test of this was performed on September 27th, 1972, by Stuart J. Freedman and John F. Clauser. Although the experiment they performed was a somewhat more primitive version than the modern experiments, it was monumental for demonstrating that there is, in fact, a difference in predictions between an interpretation that was deterministic, local, and real for what we view as quantum uncertainty compared to the standard quantum predictions.
You set your polarizer to various angles, send many sets of entangled pairs down, and you measure their polarizations. The ratios of the four possible outcomes (+ +, + -, — +, and — -) allow you to measure how correlated or anti-correlated these entangled pairs are. As the experiments showed, quantum mechanics is correct, and the variants advanced by Einstein and his adherents were not.
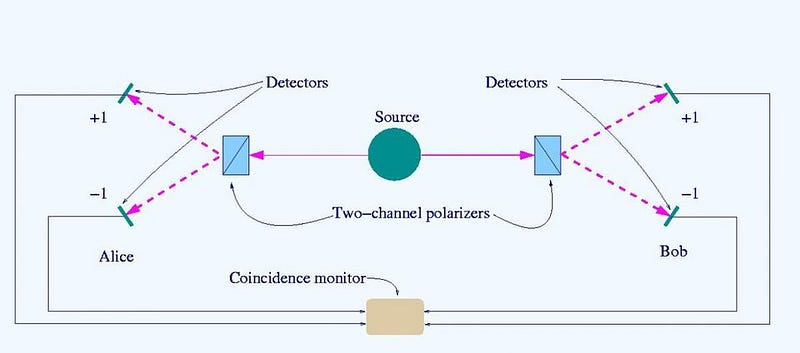
Yes; even your greatest scientific heroes can be wrong, and they can be spectacularly wrong as well. Scientific ideas are evaluated on their merit alone, not by the clout of who put them forward.
To date, all tests of quantum mechanics have been consistent with the ordinary quantum predictions, and not a deterministic variant. Confidences have been increased to over the 99.999999% level that local hidden variables are ruled out, and any behind-the-scenes physics must be incredibly counterintuitive to explain the Universe we see today.
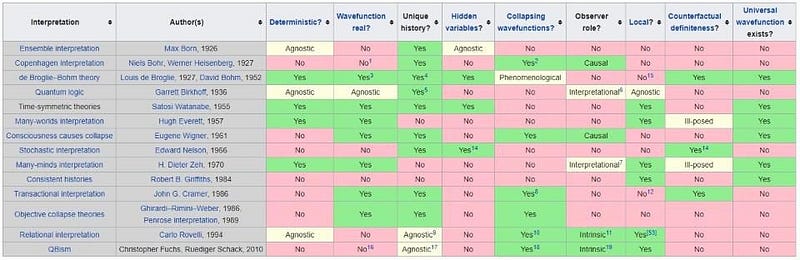
But quantum physics itself is counterintuitive. Speaking in 1985, Bell himself discussed a possible way to have a Universe governed by hidden variables, known today as superdeterminism:
There is a way to escape the inference of superluminal speeds and spooky action at a distance. But it involves absolute determinism in the universe, the complete absence of free will. Suppose the world is super-deterministic, with not just inanimate nature running on behind-the-scenes clockwork, but with our behavior, including our belief that we are free to choose to do one experiment rather than another, absolutely predetermined, including the ‘decision’ by the experimenter to carry out one set of measurements rather than another, the difficulty disappears. There is no need for a faster-than-light signal to tell particle A what measurement has been carried out on particle B, because the universe, including particle A, already ‘knows’ what that measurement, and its outcome, will be.
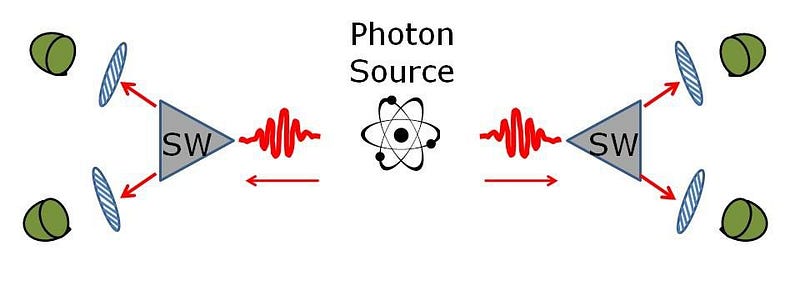
Quantum mechanics is one of the most philosophically profound and counterintuitive ideas that humanity has ever encountered. It has stood the test of time not because of its beauty, elegance, or the compelling nature of the theory, but rather because its results agree with experiment. Quantum physics was only reluctantly embraced by a great many scientists because of how divorced its rules are not only from our own experience, but of one of the great ideas of science: that we can learn the rules of nature to make accurate predictions about our own future. There’s a fundamental limit to our predictive ability, and quantum physics is what dictates that limit.
It is not the job of physics to make you comfortable with the Universe; its role is to describe reality. In that, quantum physics is an unparalleled success. But philosophically, what Bohr said all those years ago, “Anyone who is not shocked by quantum theory has not understood it,” is still true.
Ethan Siegel is the author of Beyond the Galaxy and Treknology. You can pre-order his third book, currently in development: the Encyclopaedia Cosmologica.