Could Dark Energy Wind Up Destroying The Universe?
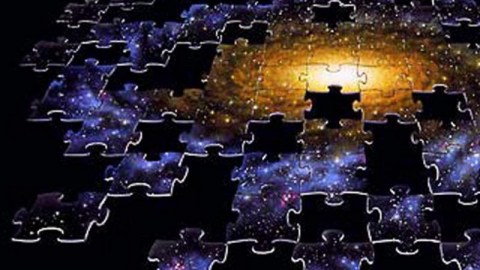
Our ultimate fate depends on something we’ve barely begun to measure.
One of the biggest mysteries in all of physics is dark energy. According to our best observations, the Universe cannot be made of matter and radiation alone, but requires an additional component that isn’t like anything else we know of. It doesn’t clump or cluster; it doesn’t have an associated particle or quantum that we know of; it appears to be the same everywhere, at all times, and in all directions. Although we can describe how it affects the Universe and place constraints on behaviors it doesn’t exhibit, we still don’t know exactly what dark energy is.
It’s eminently possible that dark energy is mundane: a form of energy inherent to the fabric of space itself that never changes, evolves, strengthens, weakens, or does anything different than what we observe it doing today. But there’s always the possibility that dark energy is more complex than the simplest, most naïve entity that we typically assume is responsible. Until we better understand what dark energy is and how it works, we have to accept an extraordinarily uncomfortable possibility: that dark energy might wind up destroying the Universe, after all.
If you want to understand what dark energy is and how it works, the simplest place to start is the very beginning of our Universe as we observe it: the hot Big Bang. At the earliest instant of the Big Bang, space was incredibly hot and dense, full of matter, antimatter and radiation at enormous energies. But it was also expanding incredibly rapidly, at just the right initial rate to balance out the attractive effects of gravity. Think about it as you would a race, with all the different forms of energy working to attract one another gravitationally, while the initial expansion drives everything rapidly apart. The Big Bang was the starting gun of this race, and you can imagine right away that there are three ways this race can end:
- gravitation wins, overcomes the expansion, and causes the Universe to recollapse in a Big Crunch,
- the expansion wins, as gravitation cannot pull things back together, and structures fly apart, ending in a Big Freeze,
- or the two balance perfectly, as the expansion rate drops to zero but never recollapses, a “Goldilocks” (or critical) situation.
How could we know the ultimate fate of the Universe? It’s very simple and very complicated at the same time. From a theoretical perspective, the Universe’s expansion rate must change over time, governed by the laws of General Relativity. As the Universe expands, it gets less dense, and it also cools as radiation loses energy. Therefore, we can learn how the Universe expands just by measuring how severely shifted the light from galaxies are, at different distances, by the expansion of the Universe.
Imagine that you could watch a single galaxy throughout the history of the Universe. At first, it would be receding very rapidly, but then it would appear to slow down as the expansion rate of the Universe dropped. Whether the Universe recollapsed or not would depend on how that apparent recession speed slowed over time. By the late 1990s, we had enough data to piece together this information, reconstructing what would happen to one galaxy from our observations of many. But what they showed was shocking: not only were all of these scenarios invalid, but distant galaxies aren’t slowing down in their recession from us, but rather are accelerating instead.
If you could have observed a single galaxy from the beginning of the Big Bang, you would have seen it recede from our perspective incredibly rapidly, then slow down for more than 7 billion years in its recession from us, moving away slower and slower as gravitation worked to counteract the expansion. And then, about 6 billion years ago, that galaxy would have stopped slowing down, switching to accelerating away from us once again.
The only explanation that is both consistent with General Relativity and the full suite of data we’ve collected is this: there’s an additional component to the Universe, even beyond dark matter, normal matter, neutrinos, radiation, black holes, and gravitational waves. It doesn’t evolve very much over time, and only becomes important when the matter-and-radiation density drops below a critical value. As far as we can tell, it behaves as though it’s a form of energy inherent to the fabric of space itself: dark energy.
If dark energy is what it seems, that has some tremendous and profound implications for the future fate of our Universe. Based on its current behavior, we’re tempted by the data to infer that dark energy is a constant: its energy density doesn’t change with time. That means, as the Universe expands and its volume increases, it actually gains energy. (Violating the conservation of energy, yes, but energy is not conserved for the expanding Universe.)
If this is truly the case, then the far future of our Universe is straightforward. Objects that were already gravitationally bound to one another some 6 billion years ago, just before dark energy began dominating the Universe’s expansion, will remain bound together, so the Milky Way and even the Local Group are safe. But structures on larger scales will continue to expand away from one another, and they’ll appear to recede faster and faster as time goes on.
Eventually, our Local Group will merge into one giant galaxy, as will all the other galaxy groups and galaxy clusters, while they all mutually expand away from one another. As time goes on, they’ll speed up so that even if we sent a spacecraft at the speed of light, they’d never reach our destination. Surprisingly and disconcertingly, this has already happened for every galaxy in our Universe that’s more than 18 billion light-years away, an estimated 94% of the galaxies in the observable Universe.
If dark energy is truly a constant, our Universe will end in a Big Freeze, and our fate will be a cold and lonely one. Unlike the “expansion defeats gravitation” scenario we talked about at the beginning, however, the expansion wins decisively, and the win gets more decisive the longer we wait. There are two plausible reasons for dark energy to behave in this fashion, and we don’t know which one (if either) is correct:
- Cosmological constant — In General Relativity, you can add a “constant” to the theory that affects the Universe’s rate of expansion always. If this constant is positive and non-zero, it could easily be responsible for dark energy.
- The zero-point energy of the quantum vacuum — In Quantum Field Theory, the lowest-energy state of a system, known as the ground state, doesn’t have to be zero, but can be a finite, non-zero value. If the ground state of empty space has a positive, finite value, that could be responsible for dark energy as well.
But there’s no inherent reason to restrict ourselves to these simple but unimaginative scenarios. In fact, if we think about the idea of energy-inherent-to-the-fabric-of-space, there’s another time in the Universe’s history where that effect should have been important: during cosmic inflation, which preceded and set up the hot Big Bang. Inflation stretched the Universe flat and gave it the same properties everywhere, and only came to an end when — somehow (we don’t know exactly how) — the energy that was formerly inherent to the fabric of space was transferred into particles and radiation, initiating the hot Big Bang.
For more than 20 years, people have speculated about a possible link between inflation and dark energy, with theories that combine the two known as “quintessence,” the original name given to “the fifth element” back when the other four were earth, fire, water and air. Today, there are four fundamental forces: gravitation, electromagnetism, and the strong and weak nuclear forces. The possibility that there’s a fifth fundamental force, and that it inflated and accelerates the Universe, is the modern incarnation of this quintessence idea.
The big difference between a cosmological constant or zero-point energy interpretation of dark energy and a quintessence interpretation is that the former two do not change over time, while quintessence can. In fact, if the big idea of quintessence is correct, it necessarily means that it did change at least once: from just before the end of inflation until the start of the hot Big Bang. And if it changed once and it isn’t in a completely stable state today, it might change yet again.
It’s that possibility for change that throws a murkiness into any attempts to predict our far future. If dark energy isn’t a perfect constant forever and ever, then any conclusions we draw about the Universe’s ultimate fate will change if that assumption proves to be incorrect. While we’ve placed fairly good constraints on how constant we know dark energy to be, they’re only good to about ~10%. NASA’s upcoming Nancy Grace Roman observatory — a wide-field, high-powered version of Hubble (formerly known as WFIRST) — will improve those constraints tenfold, giving us the capability of detecting any inconstancies in dark energy down to the ~1% level.
If it is allowed to change, three fascinating possibilities emerge for how our fate might be different.
- Dark energy could spontaneously transition to a lower-energy state. This event, known as vacuum decay, would spontaneously change the laws/constants of nature, destroying the matter that we know on a subatomic level. Wherever this transition occurs, it affects everything in that space, and the transition should propagate outward at the speed of light. If it ever reaches us, it’ll destroy us without us ever seeing it coming.
- Dark energy could be slowly and gradually increasing (or decreasing) with time. If dark energy gets stronger with time, space will eventually tear itself apart, resulting in a Big Rip scenario, tearing atoms themselves apart. Alternatively, if dark energy increases in magnitude but reverses its sign (from positive to negative), the Universe will recollapse and end in a Big Crunch, after all.
- Dark energy could slowly decay away. Rather than an all-at-once change, dark energy could undergo a slow conversion into particle/antiparticle pairs or radiation, similar to how black holes will eventually decay. This could alter our fate by placing us back on the “critical Universe” path, where the expansion rate drops to zero as the last of our dark energy decays away.
It’s really a remarkable fact that, despite all the different ways we’ve come up with to measure the farthest-flung reaches of the Universe, it still all adds up in one consistent picture. Take the laws of physics that we know, start at the beginning, and add in the right ingredients at the moment of the hot Big Bang — normal matter, dark matter, radiation, neutrinos, and dark energy — and you’ll get the Universe we observe. Despite an enormous plethora of independent ways to measure the cosmos, this one picture remains a valid explanation for each and every one.
But that doesn’t necessarily mean that dark energy is the simplest thing we can ascribe to it. It doesn’t mean that dark energy is a simple, unchanging constant, and that there’s a certain value that it has had for 13.8 billion years, and that value will never change. Instead, dark energy can take on a whole wide range of properties, and if we want to constrain what it can or cannot be, we need superior data and measurements. The future of the Universe can be known, but only with the confidence that the full suite of our observations can support. Until they get good enough to rule these alternative possibilities out, we cannot rule out that dark energy may wind up causing the Universe’s ultimate undoing.
Starts With A Bang is written by Ethan Siegel, Ph.D., author of Beyond The Galaxy, and Treknology: The Science of Star Trek from Tricorders to Warp Drive.