Ask Ethan: Why Can’t Our Telescopes Find Planet X?
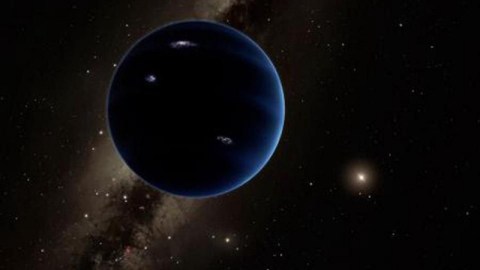
We can explore the farthest reaches of the Universe, but can’t even complete our own cosmic backyard.
The history of astronomy has been a history of receding horizons. The invention of the telescope took us beyond our naked-eye capabilities, to millions (and later billions) of stars within our own Milky Way. The application of photography and multi-wavelength astronomy to telescopes brought us beyond our own galaxy, to the distant “island Universes” populating all the space we can access. Yet, for all we know about the distant Universe, there still may be undiscovered worlds in our own Solar System. Why is that? Joseph Cummens wants to know, asking:
If scientists can use telescopes to hunt planets, galaxies, exoplanets, etc., then why can’t we scan our solar system for the elusive Planet X or other celestial bodies within our home system?
As far as we’ve peered into the Universe, we still have a long way to go, even in our own backyard.
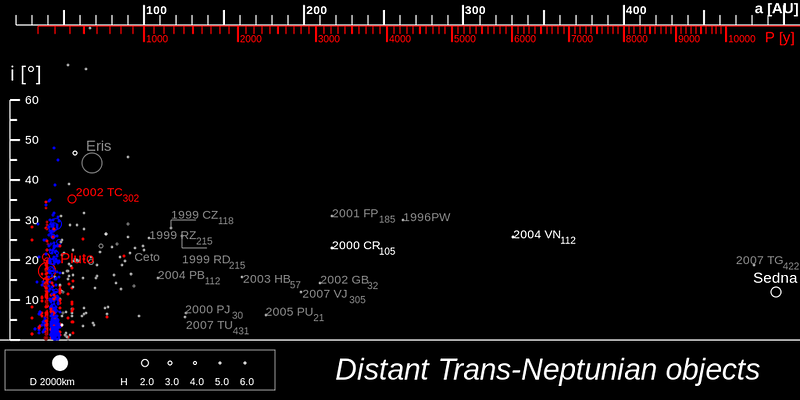
There’s a key word you need to understand that puts the entire question into perspective: magnitude. From an astronomical perspective, every object has an intrinsic brightness to it, defined by the amount of light it gives off. For an object like our Sun, this is due to its own luminance, since the Sun creates its own energy and emits it in all directions. For an object like our Moon, this is due to its reflected luminance, since it only reflects the light from other objects. The Moon has no self-luminance of its own.
If you look at the Moon during its crescent phase, you can actually make out the signal from the lunar surface that isn’t illuminated by the Sun. This isn’t some trick of the Moon’s atmosphere (since it has virtually none), but rather is due to Earthshine: sunlight reflected off of the Earth and onto the Moon.
The difference in brightness between these examples showcases how extreme the difference between reflected luminance and self-luminance are.
But there’s another thing that’s exemplified by the extreme brightness differences between the Sun and Moon, and the Moon and everything else in the night sky. The Moon has no right to appear brighter than every star, planet, or galaxy in the sky based on its own pitiful magnitude. Intrinsically, the Moon is the faintest object visible with the naked eye from anywhere on Earth. Yet it appears brighter than everything except the Sun!
The reason for this is that the Moon is so close, and that intrinsic brightness isn’t the same as observed — or apparent — brightness.
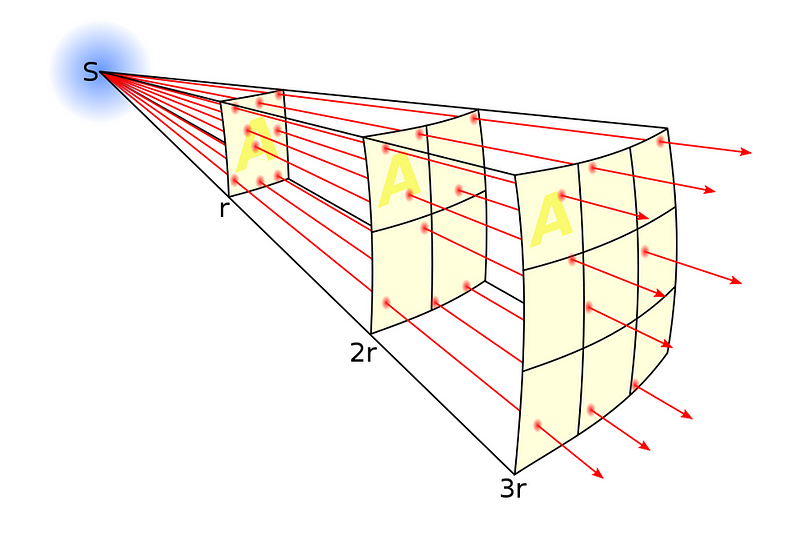
The farther away an object is, the less bright it appears. But this isn’t just some general rule we apply, there’s a quantitative relationship that allows us to determine how bright-or-faint an object appears based on its distance. Put simply, brightness falls off as the inverse of the distance squared, or b ~ 1/r².
Place an object twice as far away, and it will appear one-fourth as bright. Place it ten times as distant, and it appears just one-hundredth as bright. And place it a thousand times as far from you as it started, and it will appear just one-millionth as bright as it was initially.
For any object that emits its own light, these two factors determine an object’s apparent brightness: the intrinsic brightness and the distance it is from the observer.
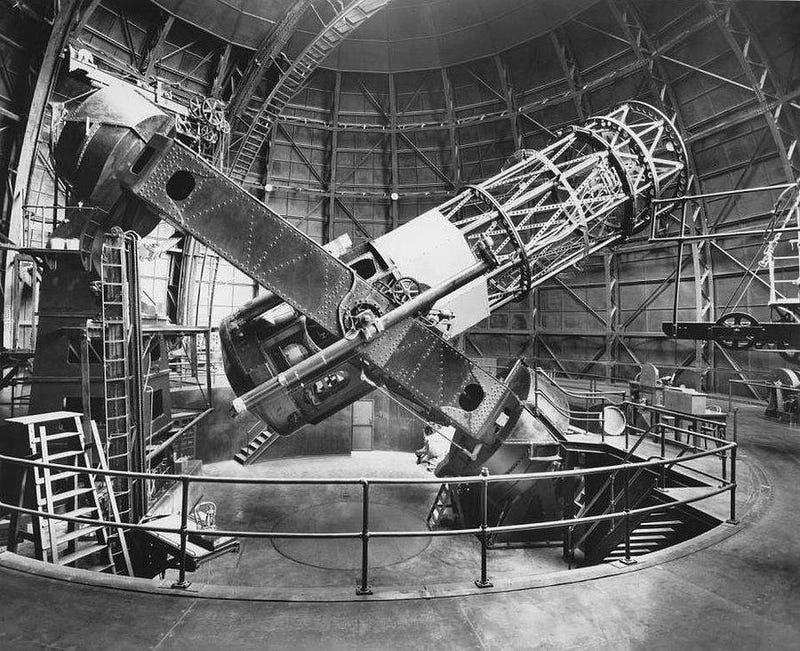
These two factors are, arguably, the two biggest ones to consider when we determine what type of telescope to build. Want to see something fainter? You’ll need to collect more light, which either means building a bigger telescope or observing the same portion of the sky for longer.
If money and engineering were no consideration, you’d opt for the bigger telescope every time. Build your telescope twice as large, and you not only gather four times as much light, but you double your resolution. To gather four times as much light by observing longer, you need to spend four times the amount of time, and gain no such advantage in resolution.
The biggest telescopes we have are capable of viewing objects to the greatest resolution possible, and resolving their details in the shortest possible time.
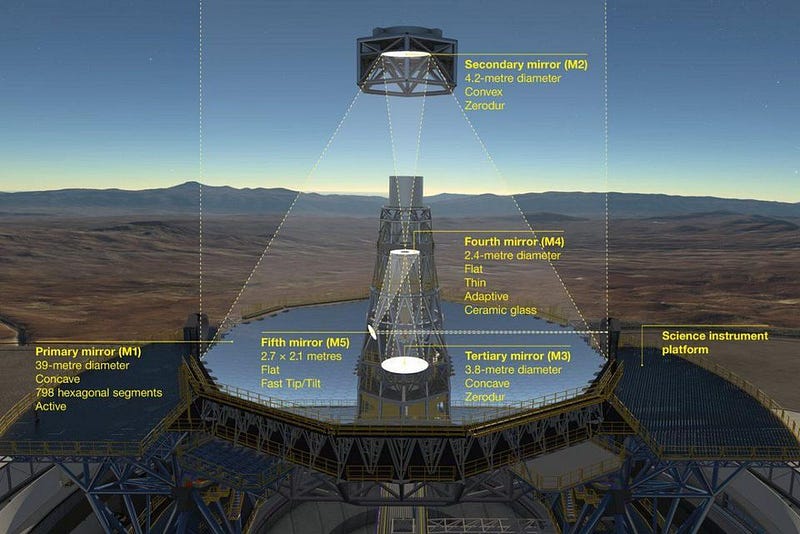
There’s also the consideration of field-of-view. What’s your goal? Is it to see the faintest object possible? Or is it to view the greatest possible amount of the Universe?
There’s a trade-off to make. Your telescope can gather a certain amount of light, and it can either do that by viewing a small region to great precision, or a large region to lesser precision. Just as a microscope can double its magnification by halving the diameter of its field-of-view, a telescope can see deeper into a region of the Universe by narrowing its field-of-view.
Different telescopes are optimized for different purposes. The trade-off is severe, however. If we want to go as deep as possible, we can only do it in one small region of the sky.
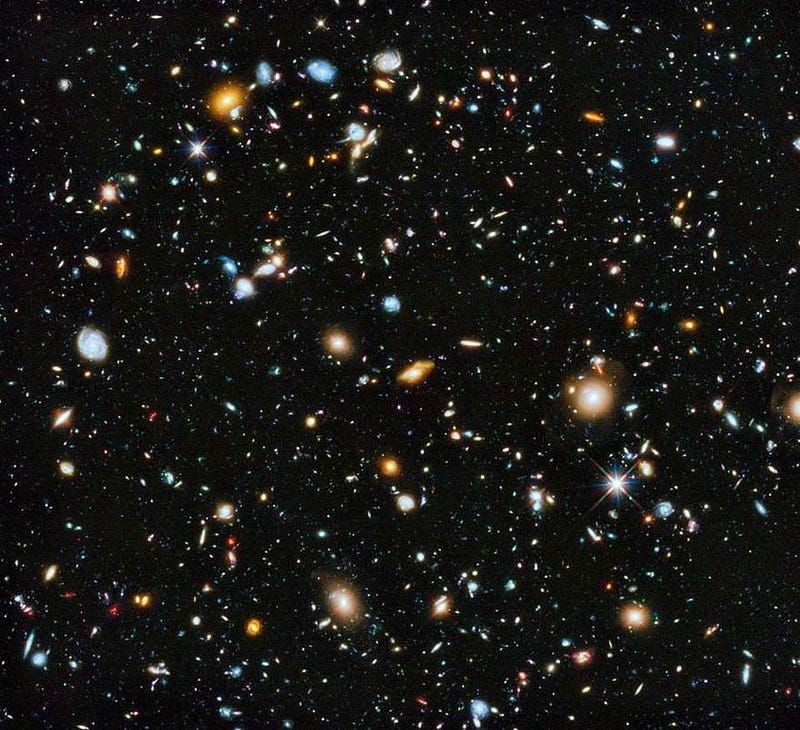
This is the Hubble eXtreme Deep Field. A tiny region of space was imaged, in a variety of wavelengths, for a total of 23 days. The amount of information that was revealed is breathtaking: we found 5,500 galaxies in this small patch of sky. The faintest objects in this patch are literally a factor of 10,000,000,000 (ten billion) times fainter than what you can see at the limit of your naked eye.
Due to its large-diameter mirror, its observations at a variety of wavelengths, its location in space, as well as its high magnification and small field-of-view, Hubble can reveal the faintest galaxies ever discovered. But there’s a cost: this image, which took 23 days of data to create, spans only 1/32,000,000th of the sky.
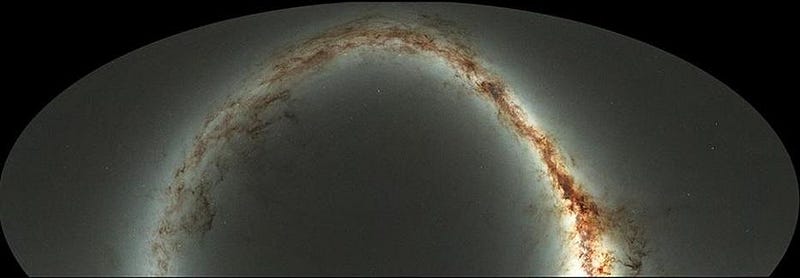
On the other hand, you can take a view like this. This was created with the Pan-STARRS telescope, which views the entire visible sky multiple times every night from its location here on Earth. It’s comparable in size to the Hubble Space Telescope, but it’s optimized for wide-field imaging, choosing to value sky-coverage over magnification.
As a result, it can reveal objects located practically anywhere on the sky; only the extreme south pole region is cut off due to the telescope’s location in the northern hemisphere. Pan-STARRS, which stands for Panoramic Survey Telescope and Rapid Response System, grabs some 75% of the sky, and is great for detecting changes between points of light. It can find comets, asteroids, Kuiper belt objects and more like no other. But it can only find objects that are thousands of times brighter than the faintest ones Hubble can detect.
As much as we’d like to, we cannot simply survey the entire outer Solar System at the required magnitude to discover everything that’s out there. A super-deep, super-faint, all-sky survey will likely never be a possibility due to technological limitations; we can go faint-and-narrow or bright-and-wide, but not both, simultaneously.
There’s also one more limiting factor that goes way back to the beginning: these objects are only reflecting sunlight. If you look to the outer Solar System at two identical objects, but one’s twice as distant as the other, it’s actually only one-sixteenth as bright. This is because by time the sunlight hits the farther object, it’s only one-quarter as bright, but then that reflected light has to travel double the distance back to our eyes, making the overall apparent brightness fall off as b~ 1/r⁴. Even if we had a Jupiter-sized world located in the Oort Cloud, we wouldn’t have found it yet.
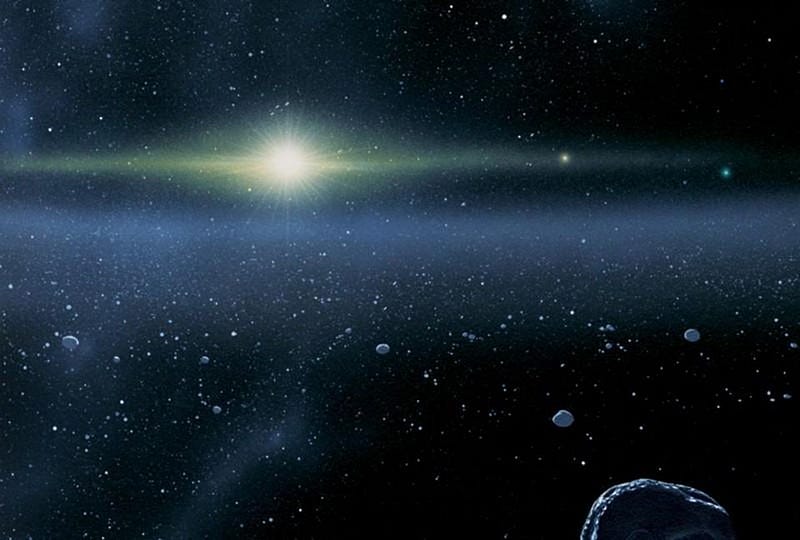
We have plenty of telescopes capable of seeing incredibly faint objects, but we need to know where to point them. We have plenty of telescopes capable of surveying huge areas of the sky, but they can only see the brighter objects; faint ones are out of reach. And for objects in our own Solar System, because they reflect sunlight rather than emit their own, self-generated light, they cannot be seen by any modern telescope if they’re located beyond a certain distance.
As with all things, the scanning we can do is powerful, interesting, and educational. It has revealed thousands upon thousands of objects within our own Solar System, from planets to moons to asteroids to Kuiper belt objects and more. But as telescope technology and sky coverage improve, we only see smaller, fainter, and more distant objects. We push the limits, but we never remove them. The science of astronomy is a story of receding horizons. But no matter how deep we go, there will always be a limit to what we can observe.
Send in your Ask Ethan questions to startswithabang at gmail dot com!
Ethan Siegel is the author of Beyond the Galaxy and Treknology. You can pre-order his third book, currently in development: the Encyclopaedia Cosmologica.