Muon mystery: New measurements challenge quantum theory
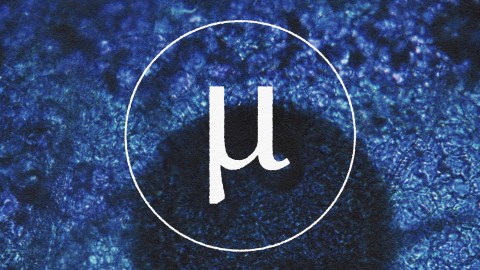
- Muons are subatomic particles similar to electrons but heavier and unstable, acting like tiny magnets due to their electric charge and spin.
- Recent research on muons reveals inconsistencies between observed magnetic behaviors and theoretical predictions, indicating the potential discovery of new physical phenomena or the need to update quantum mechanics theories.
- Resolving these inconsistencies will likely require several years of detailed theoretical work.
Science advances in a couple of ways. Occasionally, scientists will stumble upon some peculiar phenomenon that, upon investigation, reveals something very new. X-rays, radiation, and penicillin come to mind. Other advances occur because a prediction and measurement disagree — and this is the case for scientists investigating the subatomic world.
A discrepancy seen in studies of the magnetic properties of an ephemeral and unfamiliar particle called the muon might turn out to be an observation that forces scientists to rewrite textbooks on the quantum world. However, a theoretical difficulty is throwing the entire situation into question.
Unraveling muon mysteries
Muons are one example of a class of subatomic particles called leptons. The most familiar lepton is the electron, and the muon can be envisioned as the electron’s chubbier and unstable cousin. Muons are about 200 times heavier than electrons, and they live for about one-millionth of a second. Like electrons, they have electric charge and what is called “spin.” Combine spin and charge and this means that these kinds of leptons act like tiny magnets. Using the laws of 1930s quantum mechanics, the magnetic properties of leptons are predicted to have a value of 2.
In the 1940s, precise measurements of the magnetic properties of the electron revealed that they were about 0.1% higher than the simple quantum predictions. With this measurement, our understanding of the world changed. Instead of empty space being staid and placid, our world on the microscopic level is tumultuous, with subatomic particles flickering in and out of existence. A measurement of the magnetic properties of the muon soon followed, showing similar results.
In the intervening years, researchers have refined both their measurements and predictions, achieving numerical values with 10 digits of accuracy for both. However, measurement and prediction do not agree within uncertainties. A disagreement could mean a discovery, or it could mean an error in one or the other.
The first clear discrepancy was observed in measurements made using the “g-2 experiment” (pronounced “g minus 2”). It was located at Brookhaven National Laboratory, and the results were finalized in 2004. In 2013, the g-2 apparatus was moved to Fermi National Accelerator Laboratory (Fermilab), and the experiment was repeated with Fermilab’s more powerful source of muons. The Fermilab result from last year confirmed the Brookhaven result and improved on its precision. The measurement seems to be reliable.
Experiment versus theory
However, on the theory side, things are a bit more confusing. At first blush, it would seem that calculations would be straightforward: One manipulates the theoretical equations, turns the crank, and out pops the prediction. But in the case of the magnetic properties of the muon, it isn’t so easy.
The cloud of ephemeral particles surrounding the muon flitters in and out of existence, modifying the muon’s magnetic properties. The effect of many of the known particles can be calculated, but particles called quarks are very difficult to handle. Quarks are most commonly found inside protons and neutrons.
Because of the difficulty involved in doing calculations involving quarks, scientists have historically adopted a hybrid approach: They use calculations to determine the contribution for most ephemeral particles but use data drawn from other experiments to include the effect due to quarks. The data is blended with the calculations for a final prediction. The experiments that generated this data are called BaBar and KLOE.
But all of that is easier to say than do. The earlier measurements were not a perfect match for what is needed. To begin with, the earlier measurements were done at a higher energy than would be optimum for predicting the magnetic properties of muons. So, scientists needed to adjust the measurements to blend them with calculations seamlessly.
It is when these hybrid calculation/data predictions are compared with the g-2 measurements that a discrepancy is observed. Given that the two measurements agree, what is needed is a confirming prediction.
Scientists decided to use a different technique to predict the magnetic properties of muons: an all-calculation one. This approach heavily uses computers and is what is called “lattice QCD.” Using computers, researchers set up a three-dimensional grid, with a spacing of about 10-16 meters (about 1/10th the size of a proton). Using this grid, scientists can directly calculate the role that quarks play in predicting the muon’s magnetic properties. The specific calculation was performed by collaborators from three cities (Budapest-Marseille-Wuppertal), and thus is called “BMW-lattice.”
When these calculations are performed, an unsettling result emerges. The new prediction agrees neither with the g-2 measurement nor the hybrid calculation/data prediction. Instead, the lattice QCD calculations end up halfway between the two.
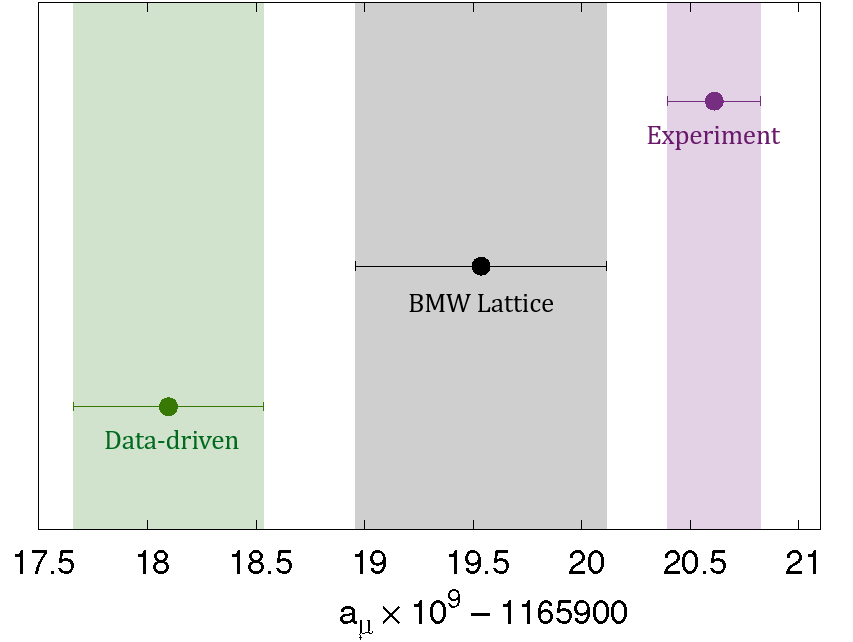
While this might seem worrisome, some caution is in order. Only one lattice QCD calculation has been performed with sufficient precision. Perhaps mistakes were made. Accordingly, other research groups are trying to reproduce this lattice QCD calculation. Until then, it would be hasty to make too much of this.
However, the siren call of a confirmed discrepancy between prediction and measurement is too powerful to ignore. So, scientists are revisiting the earlier hybrid calculation/data approach using new data from an experiment called CMD-3. The CMD-3 data is taken at a lower energy than BaBar or KLOE, and so researchers do not need to do as much manipulation to merge it with calculations.
The problem is that when the hybrid approach is used with CMD-3 data, it agrees with neither the lattice QCD approach nor the BaBar/KLOE hybrid approach. Even more confusing: CMD-3 disagrees with an earlier version of the experiment (CMD-2).
So, where does this leave us? When we compare measurements and predictions of the magnetic properties of muons, they disagree. Potentially, this could be a huge discovery. Multiple measurements of this quantity agree with each other, but there is no such agreement on the calculation side. Before any conclusion of a discovery is made, the predictions will have to tell a single and consistent story. It will take a couple of years for the work required to sort out all of the theoretical kinks.
The bottom line is that there is a lot of interest in this very precise measurement of the muon’s magnetic properties. But it will be a while before we know exactly what story it’s telling us.