The quantum egg that birthed the Universe
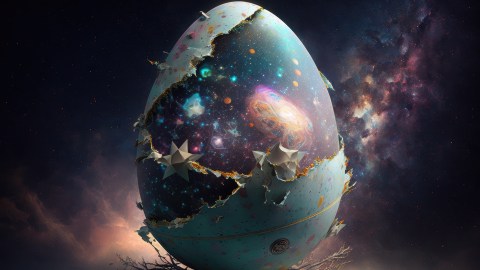
- To celebrate my 100th contribution to Big Think, there could be nothing better than to go back to the mystery of mysteries: the origin of the Universe.
- Today, we explore the ideas that seeded the Big Bang model of cosmology, a spectacularly successful attempt to describe the early history of the Universe.
- Remarkably, it all started with a cosmic egg, albeit a quantum one.
When Edwin Hubble in 1929 showed that galaxies were moving away from one another, he set the stage for a new era of cosmology. In this era, cosmologists understood the Universe to have a history — and indeed a beginning, far back in the past. That conclusion followed naturally from Hubble’s discovery: If galaxies are now moving apart (we say they are receding), perhaps there is a point in the cosmic past when they were, loosely speaking, “on top of each other,” where all matter was squeezed into a tiny volume. Pushed to its extreme, this volume becomes as small as anything the laws of physics can conceive. Of course, it is also reasonable to believe there are laws at that extreme level that we do not yet know.
Beyond space and time
Soon after, in 1931, Belgian priest and cosmologist Georges Lemaître conjectured in an article that this initial event — the beginning of the Universe — could be modeled as the decay of a single quantum of matter. One original nugget gives birth to everything else. Said Lemaître:
“If the world has begun with a single quantum, the notions of space and time would altogether fail to have any meaning at the beginning; they would only have a sensible meaning when the original quantum had been divided into a sufficient number of quanta.”
In Lemaître’s description, then, the initial state of the Universe was without space or time. Lemaître suggests that maybe this initial quantum was like a “unique atom.” The highly unstable atom “would divide in smaller and smaller atoms by a kind of super-radioactive process. Some remnant of this process might… foster the heat of the stars until our low atomic number atoms allowed life to be possible.” He concludes the very short article with a spectacular insight: “The whole matter of the world must have been present at the beginning, but the story it has to tell may be written step by step.”
To summarize Lemaître’s thesis, there was an initial state which lay beyond the normal description of space and time, something like a timeless quantum atom that spontaneously started to decay into smaller atoms, or quantum fragments. Time is a measure of change, and it only starts to pass as the atom decays. Space grows as the fragments spread away from their progenitor. Some heat or radiation is generated during the decay. The process evolves, moving through many steps until matter organizes into the atoms we are familiar with, eventually giving rise to life on this planet.
Forces of universal attraction
The onset of the Second World War turned scientists to other pursuits — those related to national defense and weaponry design. As the conflict unfolded and eventually ended, new knowledge from nuclear physics, used during the war for making bombs, started in the late 1930s to be applied to the study of the nuclear furnaces that power stars. In the late 1940s, scientists began to use this knowledge to reconstruct the early history of the Universe. How far back in time could physicists reach? How could they trace the way we got from there to here? That was, and still remains, the big challenge for the Big Bang model of cosmology.
In the mid-1930s, Hideki Yukawa in Japan proposed that atomic nuclei are held together by a force of nature never described previously, the strong nuclear force. This force’s attraction would have to overcome the electric repulsion protons would feel in a nucleus. How else could the nucleus of an atom of uranium hold 92 positively charged protons? And how would neutrons stay there if they had no electrical charge?
It became clear that atomic nuclei are something like balls of protons and neutrons held together by the strong nuclear force. (Nuclei are not balls at all, but the image is at least suggestive of how they work.)
At the time, it was also known that bonds between material objects break at high energy. That’s what happens when you boil water, for example, and liquid turns to steam. At higher energies still, a water molecule breaks into two atoms of hydrogen and one of oxygen. Push the energy high enough, and you can break the atoms themselves, separating the electrons from the nucleus. Finally, even the nucleus falls apart, separating into free protons and neutrons. The forces that keep matter together can be sequentially overwhelmed with increases in energy — which in practice means increases in the intensity of collisions between bits of matter and of radiation.
The stage was set to match this concept of sequential breaking to the history of the Universe — a Universe that began in some sort of idealized quantum state before breaking into the stuff we are familiar with, like atomic nuclei, and later, atoms.
What will become the Big Bang model, born from the pioneering work of George Gamow, Ralph Alpher, and Robert Herman in the late 1940s and early 1950s, emerges from a few core ideas: The young Universe was denser and hotter. For this reason, matter was broken down to its smallest constituents early on. It started to take shape and condense into more complex structures as time advanced and as the Universe expanded and cooled. From that uncertain beginning, it is a matter of wonder that in the long march of time, stars and galaxies, planets and moons, black holes and humans came to be.