A new theory for how oceans form on other worlds
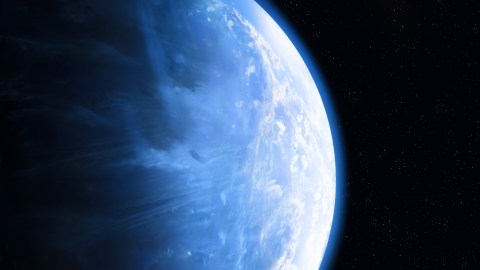
- The discovery of exoplanets has revised our understanding of water origins on planets, challenging earlier beliefs held by astronomers.
- Powerful lasers enable scientists to study the conditions inside the most common types of planets: super-Earths and sub-Neptunes.
- Innovative modeling suggests that planets may form oceans through internal processes rather than external impacts, a theory spurred by the study of exoplanets.
The most dramatic scientific progress occurs when a new window into the Universe gets thrown open. When I was a graduate student, for example, I was taught that Earth was born hot and dry. Any water present at our planet’s formation got boiled away. So, because the only window we had on planetary evolution was our own Solar System, most astronomers were sure that the water making up our oceans must have been delivered later in Earth’s history through impacts with H2O-rich comets.
But then we discovered exoplanets orbiting distant stars. A new window into the Universe was opened as, suddenly, there were lots of new planets to consider. Most importantly, astronomers were finding entirely new classes of planets that do not exist in our Solar System. Now, through the study of those truly alien worlds, a new story of planets and their reservoirs of water may be emerging.
For the past few years, I have been part of a large-scale National Science Foundation effort to study high-pressure conditions inside planets. The Center for Matter at Atomic Pressures (CMAP), based at the University of Rochester, uses the world’s most powerful lasers to squeeze small samples of hydrogen, helium, silicon, or iron as tightly as occurs deep in planetary interiors. It’s an approach that’s particularly important now that we’ve found that super-Earths and sub-Neptunes are the most abundant kind of planet in the Universe. With so much mass, the gravitational force bearing down on material inside these worlds can push our understanding of matter past the standard approximations. That’s why experiments with the giant lasers are needed.
But experiments alone aren’t the only challenge that CMAP researchers take on. Understanding exoplanets also requires new ideas and new theories. That’s why a group of us, led by two brave young postdoctoral scientists named Jisheng Zhang and Akash Gupta (both at Princeton), are working to build new computer models of exoplanets. Using these tools, we hope to help put both experimental data and telescopic observations in their proper context.
Naked worlds
The big issue we face is that many super-Earths and all sub-Neptunes begin their lives with significant rocky cores surrounded by extended atmospheres made of hydrogen and helium gas. But these atmospheres may not last. For planets that are close enough to their stars, intense UV and X-ray stellar radiation can, over time, heat the thick vails of atmospheric gas to temperatures of 10,000 K or more, driving material off into space in a massive wind. If these “photoevaporative winds” last long enough, they can strip off the entire hydrogen-helium envelope, leaving behind a bare and rocky core. Even without high UV radiation, the planet might lose its initial hydrogen atmosphere simply because the element is so light that it eventually escapes the planet’s gravity.
Because we don’t have an example of these kinds of planets in our Solar System, astronomers don’t really know what’s possible with such naked worlds. After all, the surfaces of these planets must have been under tremendous pressure sitting at the bottom of a deep, massive atmosphere. With such high pressures also come high temperatures. So, once the atmospheres are lost, the surfaces must cool down (and most likely go from a molten state to a hard crust). It’s in this transition that a new possibility emerges for the creation of oceans.
Rethinking ocean formation
Based on the modeling work of Edward Young of UCLA and collaborators, there’s much to be learned by considering the period before the atmospheres are lost. That’s when hydrogen-helium gas at high pressures is in contact with the high-temperature molten rocky surface. Until the discovery of super-Earths and sub-Neptunes, no one had much reason to explore chemical exchanges that can occur between these systems. But Young’s work found that, through these exchanges, enormous quantities of hydrogen can be absorbed into the rocky parts of the planet. Eventually, after the initial atmosphere is lost, water formed via this absorbed hydrogen can be released back into a secondary atmosphere, which then condenses, raining back down to create a planet’s census of water.
In their work, Young and collaborators suggest this process is what gave Earth its oceans (since Earth also began with a hydrogen rich atmosphere). No comets or asteroid impacts are required. In our work at CMAP, we are hoping to build new models that couple the evolution of planetary interiors with atmospheres to explore Young’s idea and go further into new territory. Personally, I find it very exciting. There’s a new, bold, and iconoclastic idea for forming oceans. What matters even more is it’s the kind of idea that might never have gotten traction if we didn’t have all these exoplanets spurring us to explore new possibilities. And that is what happens when a new window on the Universe is thrown open.