The geometry of your brain shapes its function
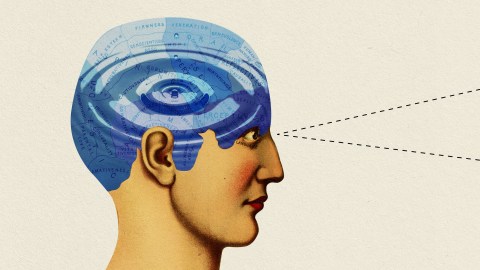
- Researchers have challenged the conventional “connectomic” view of the brain, suggesting that brain function may be more akin to ripples in a pond than signals in a telecommunication network.
- They found that their wave model, which uses information about the brain’s shape, predicted activation patterns more accurately than neuronal connectivity data.
- While the study suggests a paradigm shift in understanding brain function, critics argue that the study doesn’t consider the local brain activity patterns evoked by simple stimuli.
Modern neuroscience is dominated by one idea, the roots of which can be traced back to the mid-19th century. Around this time, behavioral neurologists began to link speech and various other functions to specific regions of the brain. Subsequently, Santiago Ramón y Cajal formulated the Neuron Doctrine, and Korbinian Brodmann published his pioneering brain map, which subdivided the cerebral cortex into 52 regions based on their cellular architecture.
Gradually, the idea emerged that the brain consists of discrete regions containing populations of functionally specialized cells, which are organized into connected networks that interact with each other via short- and long-range nerve fibers. This “connectomic” view of the brain is reinforced by widely used neuroimaging techniques that visualize the brain regions activated during cognitive tasks, as well as by the white matter tracts connecting them.
Brain physics
The connectome approach depends on abstract anatomical representations that do not take physical properties such as geometry and topology into account. But we know from physics and engineering, however, that these things do matter. For example, system dynamics are constrained patterns of motion in which all the parts of a system oscillate with the same frequency. One example is the sound made by a violin string, which is determined by its length, density, and tension.
Recent evidence suggests that these resonant wave patterns — known as “eigenmodes” — also play a role in shaping brain activity. Now, a study by researchers in Australia identifies a hitherto unrecognized role for how the geometry of the brain shapes its function, challenging the connectomic view with evidence that eigenmodes determine how activity spreads across its surface.
James Pang of Monash University and his colleagues set out to test how well eigenmodes based on brain geometry can predict brain activation and resting state activity patterns in neuroimaging studies, compared to those based on human connectome data. The researchers used a mathematical model to calculate how brain waves propagate across the wrinkled surface of the brain, and examined 10,000 brain activity maps obtained from thousands of individual neuroimaging experiments in which people performed a wide variety of cognitive tasks.
Their models showed that most of these activity maps were associated with patterns of activity distributed throughout almost the entire brain. Instead of being localized to discrete regions that spread according to connectivity, the patterns were more accurately described as wavelike activations.
Pang and his colleagues also made computer simulations of the brain’s electrical activity using a simple wave model that is used to study physical phenomena such as earthquakes. This also predicted activation patterns more accurately than connectivity data, despite only using information about the shape of the brain to constrain the wave movements.
A new paradigm in neuroscience?
The researchers say that their findings challenge the conventional view of how the brain works, which focuses largely on the transmission of signals between discrete, specialized regions. Large-scale brain function should, they say, be studied in terms of excitation waves traveling across the brain — more like ripples in a pond than signals in a telecommunication network.
“This result challenges the classical assumptions that tasks elicit focal, isolated clusters of activation,” tweeted senior author Alex Fornito, and suggests that brain activation is “dominated by low-frequency brain-wide patterns with wavelengths [greater than] 60 mm.”
David Van Essen of Washington University in St. Louis is unconvinced and says the connectome data used in the study has well documented drawbacks, and that the study authors should have incorporated local brain activity patterns evoked by simple stimuli. “It is extremely unlikely that the traveling wave model could replicate such patterns,” he told Nature News.
Pang says it would be interesting to test their model with such patterns, and that their new study merely provides proof-of-principle that brain geometry can shape brain activity.